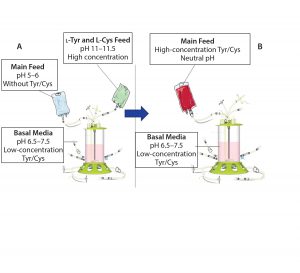
Figure 1: Traditional feeding strategies rely on a main feed and a separate alkaline feed containing l-tyrosine and l-cysteine (A). This study demonstrates the use of a combined main feed with high concentrations of tyrosine and cysteine derivatives at neutral pH (B).
Chinese hamster ovary (CHO) cells commonly are used to produce recombinant proteins such as monoclonal antibodies (MAbs) for research, diagnostic, and therapeutic purposes. Culture processes typically rely on a fed-batch approach in which a basal medium enables initial cell growth. Concentrated feeds are used to prevent nutrient depletion, thereby extending culture duration and improving cell growth, viability, and protein titer.
A neutral pH feed is desirable because culture pH should remain stable after feedings. The extremely low solubility of l-tyrosine and the low stability of l-cysteine at neutral pH have necessitated processes that leverage a slight acidic main feed and a separate alkaline feed containing these essential amino acids. Use of separate feeds at different pH levels creates the need for complex control strategies to minimize pH spikes during feed additions. In bioreactors, the alkaline feed can be added slowly while pH is monitored continuously and proportional-integral-derivative (PID) settings are adjusted to reduce CO2 sparging response to spikes in pH. In large-scale manufacturing, even with slow addition of feeds, precipitation is likely to occur after contact with neutral pH media, especially when residual foam is present.
Here we summarize results of in-depth studies that defined a simplified approach to fed-batch processes in largescale manufacturing using a highly soluble phosphotyrosine sodium salt and a new derivative, S-sulfocysteine, in neutral pH, highly concentrated feeds (Figure 1) (1, 2).
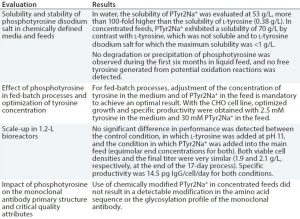
Table 1: Evaluation of phosphotyrosine disodium salt (PTyr2Na+) as a source of l-tyrosine in chemically defined, neutral pH feeds for CHO fed-batch processes
Tyrosine
Depletion of l-tyrosine in fed-batch processes has been correlated with a decrease in specific productivity and protein sequence variants (3, 4). l-tyrosine
has an extremely low solubility and is difficult to dissolve when concentrations of tyrosine disodium salts >1 g/L are used. Such high concentrations further increase the risk of media instability, mainly through coprecipitation of other amino acids. That can lead to insufficient supply of nutrients and less-than-robust processes (data not published).
To address the challenge presented by tyrosine and eliminate the need for a separate alkaline feed, we evaluated the use of a phosphotyrosine disodium salt (PTyr2Na+) as a source of l-tyrosine in chemically defined, neutral pH feeds for CHO fed-batch processes. Table 1 shows our key results.
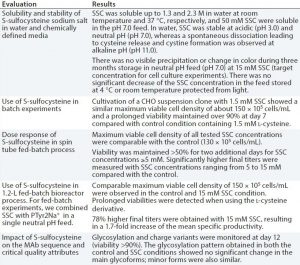
Table 2: Evaluation of S-sulfocysteine sodium salt as a source of l-cysteine in chemically defined, neutral pH feeds for CHO fed-batch processes
Cysteine
Low stability of cysteine results from dimerization and precipitation of l-cystine at neutral pH and 25 °C. This also is critical for fed-batch processes. As with l-tyrosine, an alkaline feed containing l-cysteine is used commonly, presenting similar challenges with precipitation and pH spikes. Because l-cysteine is not stable at neutral pH, we tested a new derivative, S-sulfocysteine sodium salt (SSC) in neutral pH feeds with a CHO cell line expressing a human MAb. In SSC, the highly reactive thiol group of cysteine is blocked by a sulfate group, which lowers the molecule’s reactive potential toward metals such as copper and iron and oxidation. Table 2 summarizes key results.
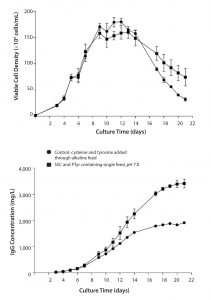
Figure 2: Comparison of culture duration and titer using a single-feed strategy with PTyr2Na+ and SSC versus a separate cysteine/tyrosine alkaline feed (pH 11); data are represented as mean ±SEM with n = 4 replicates for each condition.
Figure 2 compares the single-feed strategy using PTyr2Na+ and SSC to a fed-batch process using the classical separate cys/tyr feed at pH 11. Results show a longer culture duration and a doubling of specific productivity with the single-feed strategy.
We also explored possible mechanisms leading to the increased titer when using SSC. Intracellular labeling with carboxy-H2DCFDA demonstrated a decrease in reactive oxygen species (ROS) in the cells obtained in the SSC-containing process, pointing out to antioxidative mechanisms.
We performed microarrays on mRNA of CHO cells extracted at different time points in the fed-batch process. The gene showing the highest upregulation in the SSC condition compared with the control coded for SOD-2. Superoxide dismutases catalyze conversion of superoxide radicals to H2O2 and O2 and are known to decrease intracellular ROS levels (5). The enzymes may be partly responsible for the lower intracellular oxidative potential observed in CHO cells.
Cysteine is a key sulphur source and is the limiting factor for synthesis of glutathione (GSH), the main intracellular antioxidant. Although microarray studies showed no change in expression of enzymes involved in GSH synthesis, CHO cells cultured in the presence of SSC had an increased pool of total glutathione. That may help explain their longer culture duration as a result of several described mechanisms (6–8). The higher intracellular free glutathione pool may be linked to increased protein folding in the endoplasmic reticulum of those cells, where an oxidizing environment is necessary to add disulfide bonds into the protein (9). That can result in a higher secretory rate — and ultimately, a higher titer.
Finally, one main advantage of SSC is that the highly reactive thiol group of cysteine is protected, thus limiting the interaction of the molecule with other cell culture media components such as metals. SSC also is less susceptible to oxidation than cysteine. This contrasts with the most commonly used cysteine derivatives or prodrugs such as GSH, cysteamine, or N-acetylcysteine, in which the highly reactive thiol group is free to interact with other media components.
Advantages
MAb manufacturing depends on high-concentration nutrient feeds, which can lead to high titers and sustain specific productivity throughout the culture. Use of phosphotyrosine disodium salt and S-sulfocysteine sodium salt as components of chemically defined, neutral pH, highly concentrated feeds provides an alternative to the commonly used separate cys/tyr alkaline feed. The advantage of the approach summarized here is that it can be incorporated into existing strategies to simplify fed-batch processes in large-scale manufacturing and improve overall process performance.
References
1 Zimmer A, et al. Improvement and Simplification of Fed-Batch Bioprocesses with a Highly Soluble Phosphotyrosine Sodium Salt. J. Biotechnol. 186, 2014: 110–118; doi: 10.1016/j.jbiotec.2014.06.026.
2 Hecklau C, et al. S-Sulfocysteine Simplifies Fed-Batch Processes and Increases the CHO Specific Productivity via Antioxidant Activity. J. Biotechnol. 218, 2016: 53–63; doi: 10.1016/j.jbiotec.2015.11.022.
3 Yu M, et al. Understanding the Intracellular Effect of Enhanced Nutrient Feeding Toward High-Titer Antibody Production Process. Biotechnol. Bioeng. 108(5) 2011: 1078–1088; doi: 10.1002/bit.23031.
4 Freeney L, et al. Engineering and Supporting Technologies Eliminating Tyrosine Sequence Variants in CHO Cell Lines Producing Recombinant Monoclonal Antibodies Biotechnol. Bioeng. 110 (2013), 1087–1097; doi: 10.1002/bit.24759.
5 Mannarino SC, et al. Requirement of Glutathione for Sod1 Activation During Lifespan Extension. Yeast 28 (1) 2011: 19–25; doi: 10.1002/yea.1817.
6 Marà M, et al. Mitochondrial Glutathione, a Key Survival Antioxidant. Antioxid. Redox Signal. 11(11) 2009: 2685–2700; doi: 10.1089/ARS.2009.2695.
7 Selvarasu S, et al. Combined In Silico Modeling and Metabolomics Analysis to Characterize Fed-Batch CHO Cell Culture Biotechnol. Bioeng. 109(6) 2012: 1415–1429; doi: 10.1002/bit.24445
8 Mailloux RJ, et al. Glutathionylation Acts As a Control Switch for Uncoupling Proteins UCP2 and UCP3 J. Biol. Chem. 286(24) 2011: 21865–21875; doi: 10.1074/jbc.M111.240242.
9 Kojer K, Riemer J. Balancing Oxidative Protein Folding: The Influences of Reducing Pathways on Disulfide Bond Formation J. Biochim. Biophys. Acta 1844(8) 2014: 1383–1390; doi: 10.1016/j.bbapap.2014.02.004.
Joerg von Hagen is R&D director, Caroline Hecklau is a PhD student, Ronja Seibel is a scientist, Sascha Pering is a PhD student, Alisa Schnellbaecher is a scientist, Maria Wehsling is a scientist, Thomas Eichhorn is a scientist, and corresponding author Aline Zimmer is head of R&D, advanced cell culture technology, all at Merck KGaA, Darmstadt, Germany; aline.zimmer@emdmillipore.com. Frankfurter Strasse 250, 64293 Darmstadt, Germany.