Hydroxyapatite (HA) has a long and successful history in the field of antibody purification, and it has worked well for immunoglobulin M (IgM) monoclonal antibodies (MAbs) (1,2,3,4,5,6,7,8). Applications range from initial capture to intermediate purification to final polishing. HA is best known for its superior ability to reduce antibody aggregates, but it also supports excellent reduction of DNA, viruses, and endotoxins. As IgM MAbs exhibit increasing potential in the fields of cancer and infectious disease and in stem-cell therapies, HA’s unique fractionation abilities take on greater importance. Meeting the needs of those new opportunities requires an understanding of how HA interacts with various classes of biomolecules and how such interactions can be coordinated to create selectivities that particularly support the unique requirements of IgM purification.
PRODUCT FOCUS: ANTIBODIES
PROCESS FOCUS: DOWNSTREAM PROCESSING
WHO SHOULD READ: PROCESS DEVELOPMENT, MANUFACTURING, AND ANALYTICAL
KEYWORDS: ION-EXCHANGE, METAL AFFINITY, MULTIMODAL CHROMATOGRAPHY, CAPTURE, POLISHING, DNA, AGGREGATES
LEVEL: INTERMEDIATE
HA serves as a multimodal chromatography medium. Its principal surface reactivities include cation exchange through negatively charged, surface-exposed HA-phosphate groups and calcium metal affinity through positively charged, surface-exposed HA-calcium atoms (9,10,11,12). The positive charge on the calcium atoms may be capable of mediating anion-exchange interactions, but no experimental evidence supports this hypothesis, and it seems to be overwhelmed by the stronger influence of metal-affinity interactions (13). Hydrogen bonding also has been suggested as a possible contributor to HA interactions with biomolecules (10,11,12), but it has not been investigated systematically, and no experimental evidence has been presented indicating the magnitude of its potential contribution.
Phosphoryl cation exchange with biomolecules is intuitively straightforward. It follows the same rationale as cation exchange on familiar chromatography media such as carboxy- and sulfo-based cation exchangers. Positively charged amino protein residues participate in electrostatic interactions with negatively charged HA-phosphates. These interactions can be controlled by altering salt concentration or pH. This explains the strong interaction of HA with alkaline proteins, but a given protein need not have an alkaline isoelectric point (pI) to bind strongly to HA, and strong binding is not necessarily an indication of strong cation-exchange binding. HA-calcium binding modulates the net effects of binding through HA-phosphates (13).
The mechanism of calcium metal affinity is less intuitive but known to be mediated partly through carboxyl clusters on protein surfaces, regions where two or more glutamic and/or aspartic acid residues reside in close proximity to each other. That can create the misimpression that calcium affinity represents a simple case of electrostatic binding between the positively charged calcium and negatively charged protein carboxyls, but several lines of experimental evidence demonstrate that this is not the case. Single carboxyl groups do not mediate HA binding (10,11,12). Replacement of multiple carboxyls with same-charge sulfo groups on strongly HA-binding proteins abolishes their binding (10,11,12). HA-calcium metal affinity also persists at salt concentrations far above the levels that can be survived by electrostatic interactions — for example in saturated sodium chloride (NaCl). It is equally important to appreciate that amino groups also contribute to calcium affinity just as they do with ethylene diamine tetraacetic acid (EDTA) (Figure 1). All of these phenomena highlight the distinction between metal affinity and electrostatic interactions, which is important because it explains why protein retention on HA generally does not correlate with pI. It may do so within closely related structural families of solutes but not broadly across diverse species of proteins.
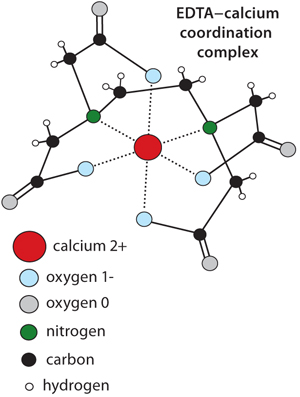
Figure 1:
HA-calcium also participates in strong metal affinity interactions with phosphate groups on DNA, endotoxins, and lipid-enveloped viruses. Such viruses interact doubly with HA: Viral envelope calcium atoms participate in metal affinity with HA phosphates, while envelope phosphate groups participate in metal affinity with HA calcium. This explains why HA supports excellent virus removal but requires extraordinary phosphate concentrations — 600 mM or higher — to elute some species of lipid-enveloped virus (14, 15). As with metal-affinity interactions between HA-calcium and protein carboxyl clusters, calcium affinity interactions with DNA, endotoxins, and virus also survive high concentrations of NaCl.
In fact, high NaCl concentrations enhance binding of phosphorylated biomolecules. Using DNA as a conceptual model, at low conductivity, HA phosphates repel same-charge DNA phosphates. This is understood to restrict the ability of DNA phosphates to interact with HA-calcium (16). The phenomenon is explained by the fact that each HA-calcium atom is surrounded by a double ring of HA phosphate residues that create an electrostatic force field interfering with the approach of negatively charged solutes (Figure 2). Increasing concentration of nonphosphate salts suppresses electrostatic repulsion and enables DNA phosphates to interact more directly with HA-calcium.
Publications up to the 1990s almost exclusively describe applying samples to HA under physiological conditions — usually in phosphate-buffered saline — and eluting with simple phosphate gradients. Buffer phosphate ions compete directly with proteins for metal-affinity interactions with HA-calcium, and the conductivity created by the phosphate salts elutes cation-exchange interactions with HA-phosphates (10,11,12). Phosphate gradients thereby prov
ide a convenient way to elute both mechanisms simultaneously, but they provide no opportunity to differentially exploit their individual contributions. Investigators began to explore these opportunities in the 1990s (9, 17) and found that proteins bound exclusively by cation exchange can be eluted by NaCl gradients, with solutes retained by metal affinity remaining bound to the column. By maintaining a low concentration of phosphate throughout a salt gradient, proteins with weak calcium affinity also elute within the NaCl gradient, and solutes retained by strong calcium-affinity interactions remain bound. Solutes bound strongly by calcium affinity are removed by higher concentrations of phosphate.
Although gradient composition provides a good start toward more effective use of HA’s potential, it represents only the beginning of the possibilities for improved IgM purification. Because the calcium affinity is resistant to elevated NaCl concentrations, neutral salts can be added directly to a sample to prevent binding of proteins that interact with HA exclusively by cation exchange. This would conserve HA surface area for binding solutes by calcium affinity. It also leaves a lesser diversity of contaminants to elute in the gradient, which translates to a cleaner IgM fraction. Alternatively, samples can be applied to HA, then washed with high salt to displace cation-exchange–bound species, after which the column can be eluted with a simple phosphate gradient or a compound gradient that includes both phosphate and NaCl.
Preelution salt washes can have unexpected value in their unique ability to dissociate otherwise-stable associations between proteins and contaminants, including between IgM and DNA. In one case, IgM eluted from HA in a phosphate gradient after a 2 M NaCl wash was shown to contain less DNA than the same IgM eluted under the same conditions but without the 2 M NaCl wash (18). The antibody remained bound during the NaCl wash through calcium affinity with HA. In another case, an IgM MAb partly coeluted with DNA in a simple phosphate gradient, whereas conducting the same phosphate gradient in the presence of 2 M NaCl caused the IgM to elute earlier because it suspended cation-exchange binding. That caused DNA to elute later because it suspended electrostatic repulsion between HA and DNA phosphates. The net result was creation of a baseline separation that substantially enhanced DNA removal (19).
DNA removal deserves special attention because recent experimental results show that IgM MAbs can form stable associations with DNA even when they have no immunospecific affinity for them (19). The DNA comes from host cells that release their nuclear contents during cell death. With higher cell concentrations and lower cell viabilities at harvest, more DNA will be present — and more aggregation will occur. Although high salt washes and elution gradients can offer better DNA than simple phosphate gradients on HA, it seems obvious that the more DNA removed before reaching that point the better.
Methods for reducing DNA content of column feed streams go back at least to the 1990s, when a number of investigators added compounds such as DEAE-cellulose or Dowex anion exchangers directly to cell culture harvests with the intent of selectively adsorbing DNA (17). Such treatment works well with IgG MAbs because most of them bind poorly or not at all to anion exchangers — especially not under physiological conditions. It encounters a limitation with IgMs because most of them do bind anion exchangers under physiological conditions (17). At pH 7.0, most IgMs elute from quaternary amino (Q, QA, TMAE) anion exchangers at 200–250 mM NaCl. Fortunately, increasing conductivity just enough to prevent IgM binding conserves a substantial ability to reduce DNA. Sample treatment with nuclease enzymes is sometimes considered for DNA reduction, but that is expensive and works poorly (19): Cell culture harvests contain salts and other components that inhibit nucleases, and most of the DNA is stabilized by its association with chromosomal debris.
Process Development PreliminariesNearly all publications recommend ceramic hydroxyapatite CHT type II media, 40 μm, for purification of IgM. This is based partly on the larger pore size distribution of type II over type I, which is understood to provide IgM with access to a larger binding surface area. For strong-binding IgMs, dynamic binding capacity on CHT type II at a linear flow rate of 150 cm/hr can be at least as high as 20 mg/mL (16). Practical experience indicates that type II media are also physically and chemically more robust than other hydroxyapatites. This is believed to derive from the ceramic formation step occurring at higher temperatures that form stronger welds between individual crystals, and it contributes to longer cycle life in manufacturing applications.
Most publications indicate that IgMs elute from HA at 150–250 mM phosphate, but unpublished studies indicate a much broader range of 50–400 mM (20). At the low end, some IgM monoclonal antibodies may not bind to HA sufficiently to achieve useful capacity. At the high end, some IgM antibodies will coelute with DNA. Some reports have shown that potassium salts support higher resolution than sodium salts for IgG applications (21), but this variable has not been evaluated systematically with IgMs.
One attraction of HA is that it supports good IgM capacity at physiological pH. Another is that the practical range for pH scouting is quite narrow. Increasing pH above neutrality generally reduces IgM retention, apparently due to reduction of cation exchange binding. It should be expected to reduce DBC as well, but this may be acceptable if the pH increase sufficiently improves contaminant removal. Upper pH limits may be imposed by the solubility and stability of a given IgM MAb, and perhaps for that reason HA performance has been rarely evaluated above pH 8.0. Reducing pH generally increases retention and capacity, but pH 6.5 represents the recommended lower limit for stability of the HA itself.
Inclusion of 5 mM phosphate is required at pH 6.5; less phosphate is required at higher pH values, but 5 mM remains a good precautionary level. Higher phosphate concentrations in samples and equilibration buffers reduce binding capacity. When using HA as a capture method, check with cell culture staff to determine the phosphate content of the culture media they’re using, and request notification of changes in media formulation. When using HA for intermediate purification or polishing, consider the buffer composition of the previous step. Besides excess phosphate potentially depressing HA capacity, citrate buffers and chelating agents can damage the HA by removing calcium from its structure. However, HA is very tolerant of surfactants, chaotropes, organic solvents, and organic polymers (1).
Linear elution gradients are generally more effective for initial scouting, but once the conditions for eluting a particular IgM have been defined, they can be converted to steps. Gradient set points should be determined after determining IgM load per unit volume of HA because loading will influence elution behavior, especially at the capture step. Determination of DBC with purified IgM generally is not useful because it cannot predict how column loading will affect contaminant reduction. To determine process capacity specifications, start at 10 mg IgM per mL of HA and then evaluate increasing loads, using contaminant content to identify the practical maximum.
Preliminary results with HA may suggest that one-step purification is feasible for some IgMs grown in protein-free media. But it is important to keep in mind that cell culture harvest composition can vary substantially with cell density and
viability at harvest, with batch variation in hydrolysates and other media components, or at different phases of cell culture process development. With preparation of IgM for in vitro applications, it will be prudent to combine HA with at least one additional purification step to absorb uncontrolled process variation arising from cell culture production. With preparation of IgM for in vivo applications, it is prudent to assume that three fractionation steps will be required.
Reduction of excess DNA is an essential prerequisite for obtaining the best IgM capture performance with HA or any other capture method. One simple, effective method uses the Dowex AG1X2 hydrophobic strong anion exchanger. To determine operating conditions, apply a sample of clarified cell culture harvest to quaternary amino-based anion exchanger at pH 7.0, then elute in a 20–column-volume (CV) linear gradient to 600 mM NaCl. The IgM peak usually will be obvious in the range of 200–250 mM NaCl, and there will be a DNA peak at the end of the gradient. Monitoring the chromatogram simultaneously at 254 nm and 280 nm helps identify DNA-containing peaks. The 254/280 ratio of pure DNA is almost exactly 2:1. The ratio is roughly reversed with purified IgMs. Determine conductivity at the IgM peak center, and adjust the salt concentration of the harvest to that value by adding NaCl.
Equilibrate Dowex AG1X2 media to the same pH and conductivity conditions, then add equilibrated Dowex particles to the harvest in an amount of 2–5% (v/v). Incubate stirring for at least an hour at room temperature, but better results will be achieved by mixing for at least four hours at ambient temperature. Overnight mixing at 4–8° C is convenient and effective. Stirring intensity should be no greater than sufficient to keep the Dowex particles suspended. Remove those particles using centrifugation and/or filtration, and rinse with equilibration buffer to maximize IgM recovery. In addition to removing a major contaminant (DNA) that interferes with HA performance, this treatment will reduce lot-to-lot variation of harvest composition and help improve reproducibility of the subsequent purification process. Figure 3 compares analytical size-exclusion chromatography (SEC) profiles of untreated and Dowex-treated IgM cell culture harvest. This clone produced about 100 mg IgM/L and was harvested at about 80% cell mortality. DNA was reduced >99% from 93,733 ppm to 692 ppm of IgM. Host-cell protein (HCP) contamination was reduced about 9%. Aggregates were reduced about threefold from 23% to 6.7%. And IgM recovery was 95%.
HA binding of most IgMs includes a substantial component of calcium affinity. This is consistent with most IgMs also exhibiting strong binding to anion exchangers, which is mediated by their high content of carboxyl groups. Even IgMs with pI values above 9 bind strongly to anion exchangers at pH 7.0 (22). This highlights that pI is not predictive of HA retention and illustrates that positive and negative charges are concentrated on different regions of the IgM. The practical significance of IgMs interacting strongly with HA by calcium affinity is that their binding is more tolerant of NaCl than ion exchange, which means that a sample often need not be diluted or buffer-exchanged to achieve good binding capacity.
Three distinct screening protocols (Table 1) may be evaluated initially. The first consists of applying harvest, washing with phosphate buffer, and eluting in an increasing phosphate gradient. The second protocol involves the same equilibration, loading, and elution steps but includes a 5–10 CV wash with 2 M NaCl, then a brief wash back to equilibration buffer before applying the phosphate gradient. That wash step is intended mainly to dissociate IgM–DNA complexes, but it typically reduces HCP contamination as well. The third protocol shares the same equilibration and loading conditions and begins with a wash to 2 M NaCl, but it differs by maintaining a high salt concentration throughout the phosphate gradient. Doing so virtually abolishes the cation-exchange component, for all practical purposes creating an exclusively calcium affinity surface.
Table 1:Each protocol can be performed on 1–2 mL columns, loading a volume of harvest that corresponds to ≤10 mg of IgM per mL of HA. A smaller sample volume can be used if supply is limited, but use enough to ensure reliability of analytical results. Applying larger amounts at this stage risks exceeding the capacity of the HA. The eluted IgM should be evaluated for aggregate reduction by analytical (SEC) and for HCP reduction by enzyme-linked immunosorbent assay (ELISA). For DNA reduction, quantitative polymerase chain reaction (qPCR) provides the most sensitive and accurate results, but intercalating fluorescent dye assays are less expensive, more convenient, and generally adequate for preliminary process development. Reduced sodium-dodecyl sulfate polyacrylamide gel electrophoresis (SDS-PAGE) can be included as well, but SEC generally provides more useful guidance, especially if cells were cultured in protein-free media.
Figure 4 provides HA chromatograms for the three screening protocols described in Table 1. The upper series shows results from cell-culture harvest clarified by centrifugation and microfiltration. The lower series shows results from clarification using Dowex ion-exchange media. Figure 5 shows the corresponding SEC results of the IgM peak for each HA experiment in Figure 4. And Figure 6 compares results from reduced SDS-PAGE. Finally, Table 2 summarizes analytical results for HCP, DNA, aggregates, and recovery.
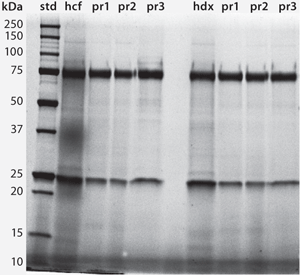
Figure 6:
Figure 4 highlights the benefits of advance DNA removal, essentially leaving a well-defined IgM peak and a modest HCP load. After achieving 99% DNA reduction by itself, the Dowex ion exchanger enabled the three screening protocols to reduce DNA by up to an additional 3 logs (Table 2). Expectedly, the lowest DNA values were associated with 2 M NaCl steps. Lacking Dowex treatment, the phosphate gradient reduced DNA by 96%, and the 2 M NaCl wash followed by a phosphate gradient reduced it by 98%. The phosphate gradient at 2 M NaCl was slightly more effective than Dowex media alone, achieving 99.9% DNA reduction, but that does not make it an attractive option. The large DNA peak in the Figure 4 chromatogram must be understood to consume binding capacity that could be have been applied more productively to binding IgM. Although the DNA is less localized in the phosphate gradients without NaCl, its effects on IgM binding capacity seem likely equivalent. PAGE showed cleaner results for all Dowex-treated samples (Figure 6).
Table 2 highlights the exceptional IgM recoveries obtained with phosphate gradients conducted at 2 M NaCl: 96% from both the Dowex-treated and untreated harvest. This has been attributed to the elution conditions more effectively dissociating subpopulations of IgM from contaminant complexes; the contaminant component otherwise causes associated IgM to elute apart from unencumbered IgM (18). Note that the DNA peak in Figure 4 is much better defined than in phosphate gradients, suggesting that it was one of the major components dissociated from the IgM. But higher IgM recovery comes at a price, reflecting the suppression of cation-exchange binding by the high salt concentration. DBC on HA equilibrated with 2 M NaCl was 10 mg IgM/mL, half the value achieved in the absence of NaCl. 2 M NaCl during elution also supported the weakest aggregate removal, with the strongest achieved by simple phosphate gradient elution after a 2 M NaCl wash.
Problems in IgM Process Development: Although the screening protocols in Table 1 generally work well, it’s wise to expect the unexpected. Some IgMs do not bind HA strongly enough to support good capacity, which may disqualify HA for an industrial process. But there are several research-scale work-arounds that can produce a good enough result to eliminate the need to develop an alternative approach. The most obvious is that purification of research quantities does not require high process economy. So use a bigger column. If you double the column size, keep the gradient volume of the smaller column to maximize eluted product concentration from the larger column.
The second work-around is to omit phosphate from the binding and wash buffers, thus permitting full exploitation of HA calcium affinity. The third work-around — reducing pH — works by enhancing cation-exchange binding. Reducing phosphate and pH should provide a bigger capacity boost than either alone — but be aware that such tactics are not supported by HA suppliers for the very good reason that both are detrimental to the stability of the media. Unless you conduct studies to document HA stability through some defined number of cycles, be prepared to retire a given column after a single day’s work. However, considering the workload associated with developing an alternative purification platform, that may be an acceptable price to pay, especially if you need the antibody today.
Another challenge can derive from some IgMs being cryoglobulins: decreasingly soluble at temperatures below 37° C, particularly under refrigeration (17, 23). Cryoprecipitation can be sufficiently strong to provide a convenient purification tool. Chill the harvest, discard the soluble contaminants, and resolubilize the IgM by warming (24). The problem is that cryoglobulins sometimes form colorless, gelatinous depositions on container walls, where they can go unnoticed. This has too frequently caused process developers to conclude that an IgM bound irreversibly to a column when the real explanation was that most of it never got there at all. The real problem is typically discovered after the original containers (still containing most of the IgM) have been discarded or washed. Warming refrigerated samples to at least room temperature before applying samples to HA is recommended for any IgM that has not been previously characterized. Bear in mind also that antibodies with severe temperature-solubility issues also can impose unique challenges on formulation developers. They may even have consequences for the intended therapeutic application.
Polishing Steps Following IgM Capture By Hydroxyapatite: SEC has been the favorite post-HA step among researchers for decades. HA performs the important job of concentrating IgM, then SEC provides excellent removal of aggregates, fragments, and HCP with virtually no need for method development. This is an especially attractive option when the IgM is eluted with a phosphate gradient in 2 M NaCl because SEC provides the additional benefit of buffer exchanging the IgM into a convenient working formulation. Prepacked analytical SEC columns support cycle capacities up to a few milligrams. Columns with diameters up to ~2.5 cm increase capacity up to ~100mg per run. SEC is disfavored for industrial-scale purification of most proteins because it is slow, has low capacity, and dilutes samples. Such problems are even more prevalent with IgM because its large size and low diffusion constant require lower flow rates to obtain good resolution. Whatever flow rate you would use for IgG, cut it in half (at least) for IgM, then evaluate even lower flow rates.
Anion exchange is a good platform polishing candidate for high-production requirements or research applications that require especially low DNA content. Most IgMs bind very well to anion exchangers. If the IgM was eluted from HA with a simple phosphate gradient, it is often possible to equilibrate the sample for anion exchange by doing nothing more t
han diluting it with water. Like HA, anion exchange is renowned for removing DNA, viruses, and endotoxins, so together the two make an especially powerful combination. Cation exchange is a worthy polishing candidate when a third step is necessary, but many IgMs do not bind strongly enough to support good capacity.
Multimodal hydrophobic cation exchangers also provide a good third-step option (25). They may help surmount the capacity limitation of traditional cation exchangers and enable loading with less — or no — dilution or pH adjustment of HA eluates. Hydrophobic-interaction chromatography (HIC) has been used to polish IgM eluted from HA (26) and may be especially suitable if IgM elutes from HA at a high concentration of NaCl. Use the ligand that supports the strongest binding that does not denature the antibody (17). The large size and low diffusion constant of IgM molecules are also impediments to adsorption chromatography methods — including HA, but not to the same extent as with SEC. A linear flow rate of 200 cm/hr represents a reasonable maximum. Process time becomes increasingly burdensome at <100 cm/hr but bears evaluation because it supports better resolution and higher recoveries.
Hydroxyapatite for Intermediate Purification or PolishingMethod development for HA as a polishing step can be performed with the same screening protocols as above. HA’s salt tolerance is a particular asset here because it simplifies sample equilibration following a previous fraction step. In addition to the elevated salt concentration that might come from a previous ion-exchange step, HA also tolerates high concentrations of polyethylene glycol (PEG), such as after a precipitation step (27) or after steric-exclusion chromatography (28). PEG flows through during HA sample application, which conveniently removes it from the process stream. If HA is the last step in a process, there is no restriction on the concentration of NaCl in the eluted IgM; it can be easily removed during diafiltration into the final formulation.
Cleaning and MaintenanceCHT type II media can tolerate very aggressive conditions for cleaning and sanitization. If a feed stream was treated to remove excess DNA, then 500 mM phosphate at pH ~7.0 will generally support adequate cleaning. NaCl must be absent at this step because it will enhance retention of phosphorylated contaminants such as DNA, endotoxins, and lipid-enveloped viruses. After cleaning, rinse columns with one or two CVs of the original equilibration buffer to reduce the phosphate concentration, then sanitize with 1 M NaOH. Doing so is most effective at low flow rates because contaminants liberated from the apatite surface are washed away rather than needing the NaOH to merely hydrolyse them in situ (as with static sanitization). Run 1 M NaOH over the column at 200cm/hr until conductivity measurements indicate that the column is fully equilibrated, then reduce the flow rate to 5–10cm/hr for an hour or more. Sanitized HA can be stored with lesser concentrations of NaOH (e.g., 0.1 M) or in 20% ethanol. In the latter case, ethanol should be formulated in a buffer containing at least 5 mM phosphate at pH 7.0.
HA can become discolored at the column inlet over time (29), which reflects binding of contaminating metal ions. Discoloration is most frequently yellow to reddish-brown, indicating iron accumulation. Common iron sources include cell culture additives, leachate from water-for-injection (WFI) systems, and leachate from the fluid-contact surfaces of buffer tanks and chromatography skids. Such discoloration is permanent, but it does not affect media performance (25), and it highlights a benefit of HA that no other commonly used purification method can match: removal of contaminating metal ions. HA accordingly eliminates a source of process variation that is uncontrolled by other fractionation methods.
Author Details
Corresponding author Pete Gagnon is group manager for the downstream processing group, which includes Sarah Maria Abdul Latiff, Celestine Cai, Wilson Lau, Chiew Ling Lim, and Hui Theng Gan, at the Bioprocessing Technology Institute, 20 Biopolis Way, 06-01 Centros, Singapore, 138668,
REFERENCES
Hydroxyapatite.
New technologies information needed.