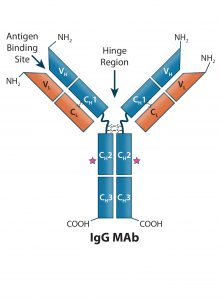
Figure 1: Human IgG1 antibody basic structure — the monoclonal IgG antibody (MAb) consists of two identical heavy chain (HC) and two identical light chain (LC) subunits. Each HC has four domains (one variable VH and three constant CH1, CH2, and CH3); each IgG LC has two domains (one variable VL, and one constant CL).
Antibody-based immunotherapy has advanced significantly since 1986, when the US Food and Drug Administration (FDA) approved the first mouse monoclonal antibody (MAb) for clinical use: Orthoclone OKT-3 (muromonab-CD3). In the intervening years, researchers have applied the tools of genetic engineering to clone immunoglobulin G (IgG) genes into a number of expression vectors. In the 1990s, the bioprocess industry was able to produce fully human antibodies in cultured cells.
As of June 2017, the FDA and the European Medicines Agency (EMA) had approved 63 and 54 MAb-based therapeutics, respectively. The global market for MAbs continues to expand dramatically, and they are now used to treat a broad range of diseases — e.g., cancer, cardiovascular disease, chronic inflammatory diseases, and viral infections — as well as for disease detection and diagnostic imaging purposes. The success of this drug class has stimulated research into novel protein engineering methods, from which has come development of bi- and multispecific antibodies.
Whereas MAbs are bivalent and monospecific (1), bi- and multispecific antibodies are designed to bind two or more unique epitopes. At this time, two bispecific antibodies have been approved as therapeutics:
- Fresenius Biotech and Trion Pharma’s Removab (catumaxomab), an IgG-like bispecific antibody (bsAb) approved by the EMA in 2009 for treatment of malignant ascites (2)
- Amgen’s Blincyto (blinatumomab), a non–IgG-like bispecific T-cell engager approved by both the EMA and the FDA in 2015 for treatment of refractory B-cell acute lymphoblastic leukemia (3, 4).
More such antibodies are in the current clinical pipeline (Table 1). In addition to other Removab indications, as Table 1 indicates, one IgG-like bsAb called emicizumab (ACE910, RG6013) has completed phase 1–2 clinical trials in Japan and is designated a breakthrough therapy by the FDA. Four more IgG-like bsABs have completed phase 1 in the United States (5).
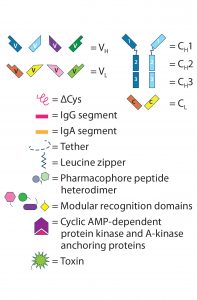
Figure 2 (key): IgG-like bsAb molecular designs use the full IgG structure as a scaffold on which to build a bi- or multivalent Ab with two or more unique specificities.
bsAb Engineering and Structural Characteristics
MAbs are heterotetrameric glycoproteins with heavy and light chains folded into discrete structural and functional domains (Figure 1). The modular domain structure of IgG molecules facilitates protein engineering, making possible the modifications necessary to create the many bi- and multispecific antibody molecular formats. Collectively referred to as “bsAbs” in this review, bi- and multispecific antibodies maintain the fundamental commonality of at least two variable binding domains that can bind two or more unique epitopes.
Like MAbs, IgG-like bsAbs can perform many different functions, including delivering a payload such as a toxin or radiolabeled compound, inactivating a ligand, acting as a receptor antagonist, and mediating antibody-dependent cell-mediated cytotoxicity (ADCC) or complement-mediated cytotoxicity (CMC). However, the dual specificity of bsAbs creates additional therapeutic options for diseases that do not respond sufficiently to monospecific MAbs. The “Functions” box lists bi- and multispecific antibody functions, and the “Advantages and Disadvantages” box lists those for bi- and multispecific compared with MAbs. Part 2 of this review will go into greater detail on the current capabilities of bsAbs.
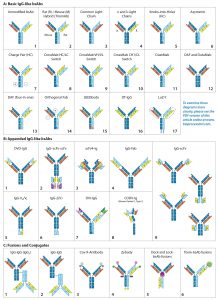
Figure 2: (Key on previous page) IgG-like bsAb molecular designs use the full IgG structure as a scaffold on which to build a bi- or multivalent Ab with two or more unique specificities. IgG-like bsAbs are categorized into three main subgroups: (A) basic IgG-like, (B) appended IgG-like, and (C) fusion/ conjugated IgG-like bsAbs. Specific structures are described in the text.
Bi- and multispecific antibodies can be divided into two broad groups: those that are IgG-like and those that are non–IgG-like. IgG-like bsAbs retain a complete IgG molecule as their main structural component. Non–IgG-like bsAbs are composed of individual IgG domains or a combination of such domains connected using short, flexible polypeptide linkers. In this review, we focus specifically on IgG-like bsAbs, which can be subcategorized further based on whether they have additional structural modifications, appending structures, nonchemical fusions, or chemical conjugations — or whether they are hybrids of IgG-like and non–IgG-like components. Figure 2 presents simplified illustrations of IgG-like bsAb formats that have been explored.
IgG-Like bsAb Formats
Creating a product with the correct combination of heavy and light chains is a major challenge in bsAb production. Originally, unmodified IgG-like bsAbs (Figure 2a-1) were created either by using a hybrid hybridoma or by recombining the reduced components of two parental IgG molecules. Both are inefficient methods for manufacturing. In both cases, heavy chains (HCs) and light chains (LCs) associate randomly to produce a large, mixed population of IgG molecules. The random assortment of heavy and light chains results in 10 potential combinations, only one of which is the correct product (both bivalent and bispecific). Of the nine other configurations, two are bivalent but monospecific, four are monovalent and monospecific, and three cannot bind either epitope. Early researchers found ways to promote the formation of and/or selectively capture the desired product. As the fields of protein engineering and molecular modeling advanced, the bsAb format designs became more divergent and ambitious.
Basic IgG-Like bsAbs: Rat/mouse hybrid TrioMAbs (Figure 2a-2) were an early form of bsAb to use preferential pairing for directing heavy- and light-chain association. Expressed by rat/mouse quadromas, the rat and mouse heavy chains (HCs) preferentially pair with light chains (LCs) of their own species. With no preference for HC heterodimerization, however, they form both HC homo- and heterodimers. Capture of the desired heterodimer product is facilitated by protein A binding affinity. Mouse HCs can be bound by protein A, whereas rat HCs cannot. As a result, the rat HC homodimer does not adhere to the resin but flows through. The HC heterodimer and mouse HC homodimer bind, but the former elutes at a higher pH than the latter, enabling purification (6). This bsAb format includes both catumaxomab and ertumaxomab (although clinical phase 2 for the latter was cancelled) (7, 8).
Common LC bsAbs (Figure 2a-3) are another type of basic IgG-like bsAb that combines a single common LC with two unique HCs to confer bispecificity. The λ/κ LC bsAbs by Novimmune (Figure 2a-4) combines two different LC classes (λ and κ) to facilitate selective capture of the final product. That capture method requires three sequential affinity steps (protein A, κ, and λ affinity chromatographies) (9).
Functions of IgG-Like bsAbs |
|
Advantages and Disadvantages |
In comparisons of IgG-like bi- and multispecific antibodies with monoclonal antibodies, the following differences become apparent: |
Advantages
|
Disadvantages
|
HC-Modified Basic IgG-Like bsAbs: To overcome those complexities, scientists have used molecular modeling and protein engineering to introduce unique structural characteristics that favor HC heterodimer formation.
Developed in 1996 by Ridgway et al. at Genentech, knob-into-hole (KiH) HC engineering (Figure 2a-5) was one of the first strategies to be implemented. The “knob” was created by substituting an amino acid located at the CH3 domain interface of one of the two HCs with a bulky amino acid. The “hole” was created by substituting the amino acid in the corresponding position of the other HC with one containing a smaller side chain. The “knob” and “hole” make formation of the homodimer sterically unfavorable and consequently promote association of HC heterodimers. Producing the “knob” half Ab and “hole” half Ab in separate cell lines and then combining the two halves can produce a desired product at high yield with limited mispairings (10, 11).
A KiH-type modification also has been engineered into CH1 and CL domains to direct HC–LC pairing. Azymetric bsAbs (Figure 2a-6) from Zymeworks, for example, use both the CH3 KiH and the CH1–CL KiH modifications.
Compatible charge pairs (Figure 2a-7) function similarly to KiH-modified bsAbs using charge repulsion instead of steric hindrance to promote heterodimer formation. Specific amino acids on opposite CH3 domain interfaces are replaced with side chains of opposing electrical charge. HC homodimers are repelled by the same charge, but heterodimers are stabilized (12).
EMD Serono’s SEEDbody technology (Figure 2a-15) uses strand-exchange engineered domains (SEEDs), CH3 domains engineered to contain alternating segments from human IgA and IgG molecules. HCs containing those domains preferentially form complementary heterodimers (13).
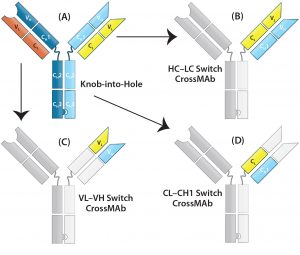
Figure 3: CrossMAb structure — the technology uses a combination of KiH to promote HC heterodimerization and domain switching between the LC and HC of a single Fab arm to ensure correct HC–LC pairing. (A) Starting with a heavy chain KiH bsAb, three CrossMAb bsAb variants were created: (B) an HC–LC switch, (C) a VH and VL switch, and (D) a CH1 and CL switch.
LC-Modified Basic IgG-Like bsAbs: CrossMAb bsAbs (Figure 2a-8, 2a-9, 2a-10) are designed to eliminate HC–LC mispairing. In this format, Schaefer et al. of Genentech used genetic engineering to switch the HC and LC domains on one of the two Fab arms into three different configurations (Figure 3). Those domain switches significantly reduce incorrect HC–LC pairing by altering the position of compatible domain interfaces on a single Fab arm (14–16).
Creative Biolabs’ LUZ-Y bsAbs (Figure 2a-17) use reversible HC and LC modification to promote correct paring. Complementary leucine zippers are engineered onto the C-terminal end of HCs to promote heterodimerization. Polypeptide tethers connect the HC’s N-terminus to the LC’s C-terminus to direct HC–LC pairing. The leucine zippers and HC–LC tethers can be cleaved using lysyl endopeptidase (Lys-C) and furin protease, respectively, resulting in a final product with only a single amino acid difference from the native molecule (17, 18).
Dual-action Fab (DAF), or “two-in-one” bsAbs (2a-12), have bispecificity engineered directly into each of their Fab arms. Because each Fab consists of six total complementarity-determining regions (CDRs), Bostrom et al. created a Fab that binds two unique epitopes by engaging different combinations of those CDRs. Each Fab arm thus recognizes two different epitopes, however because the CDRs used for antigen recognition overlap, each Fab arm can bind only to one antigen at a time (19).
Dual-target (DT) IgGs (Figure 2a-12) are similar to DAF IgGs (Figure 2a-13), in that the bispecificity is engineered into both Fab arms. In this iteration, however, the VH domains bind one antigen and the VL domains bind a different antigen. Thus, each Fab is capable of binding either one of two antigens.
Additional Modifications: Other modifications have been made to the basic IgG structure to promote formation of or to selectively capture the desired combination of heavy and light chains. For example, modification of protein A binding regions on HCs can be used to capture HC heterodimers selectively. All human IgGs bind protein A with high affinity, except for IgG3. Ablating protein A binding in one of two HC CH3 domains alters the elution pH of the HC heterodimers compared with homodimers (20).
Modifying the position of the CH1–CL disulfide bridge in one of the two Fab arms can be used to drive correct HC–LC chain pairing because incorrectly paired structures cannot form disulfide bridges and are less stable than their correctly paired counterparts (21). Several modifications can be used in combination to maximize production efficiency. For example, Medimmune’s DuetMab platform (Figure 2a-11) uses a combination of modified protein A binding, HC KiH, and a position-modified CH1–CL disulfide bridge (21); Creative Biolabs Orthogonal Fab technology (Figure 2a-14) combines HC KiH with modifications of the VH–CH1 and VL–CL interfaces, including compatible electrostatic-charge pairing (22); and the same company’s CrossMAbs (Figure 2a-8, 2a-9, and 2a-10) use a complex combination of KiH and domain switching (Figure 3) (14–16).
Appended IgG-like bsAbs contain additional antigen-binding moieties, often attached using short polypeptide linkers that consist of three or more SGGGG repeats. These antigen-binding moieties often are IgG “fragments” extending from the N- or C-termini of the LC/HC. The fragments include an isolated Fv fragment (consisting of the VH and VL Figures 2b-1, 2b-5, 2b-7, and 2b-8), an isolated VH or VL domain (Figure 2b-6) a modified version of the Fv fragment called a single-chain variable fragment (scFv, consisting of the VH and VL connected using a short polypeptide linker, Figures 2b-2, 2b-3, and 2b-9), an antigen-binding fragment (“Fab,” consisting of the VH–CH1 paired with VL–CL, Figure 2b-4), or a (Fab’)2 fragment (consisting of both Fab “arms” of an IgG antibody including the hinge region). The fragment-crystallizable (Fc) region consists of the hinge region and CH2–CH3 of both HCs and also can be engineered onto an IgG scaffold. Although addition of the Fc region does not alter valency or specificity of the resulting Ab, it may confer secondary effector functions such as ADCC, Fc receptor (FcγR) binding, and interactions with complement proteins (23, 24). It is more typically seen with non–IgG-like bsAbs. The appended IgG can be derived from a monospecific MAb with additional antigen-binding moieties attached to convert it into a bispecific Ab, or an already bispecific Ab with additional binding moieties making it multispecific.
One example of appended IgG-like bsAbs is AbbVie’s Dual Variable Domain (DVD)-IgG technology (Figure 2b-1), with two unique variable domains in tandem on each half antibody. Another is the “Morrison Format” IgG-scFv (Figure 2b-9) in which an scFv is tethered to the C-terminus of each HC on an IgG molecule to produce a quadrivalent, bispecific antibody (25).
Fusion or Conjugated or Component Fusion IgG-Like bsAbs: Conjugated bsAbs are chemically conjugated to other molecules, such as another IgG molecule (Figures 2c-1 and 2c-2) or a small molecule (Figures 2c-3, 2c-4, and 2c-6. The purpose might be either to confer additional specificities, transport a payload, or increase plasma retention. Examples include Zybodies (Creative Biolabs, Figure 2c-4), which use modular-recognition domains (MRDs) conjugated to the N- and/or C-termini of HC and LCs of a bsAb, and CovX-bodies (Pfizer, Figure 2c-3), which use a scaffold MAb as a base and covalently link nonAb-based peptide-binding heterodimers to the scaffold (26).
Component-fusion bsAbs, on the other hand, are genetically engineered. The individual components are produced separately and then assembled without chemical crosslinking. The Dock-and-Lock (DNL) format (Figure 2c-5) exploits two protein-binding domains of the cyclic 5′ adenosine monophosphate (AMP)–activated protein kinase known as PKA. Those domains allow for stable dimerization of PKA and binding to the A-kinase anchoring protein (AKAP). By linking PKA or AKAP domains to the C-terminus of an antibody, Fab, (Fab’)2, or scFv and producing the DNL-fusions in separate cell lines, the DNL concept can be used to combine many different binding moieties to produce a wide variety of multivalent, multispecific products (27).
Hybrid IgG-Like/Non–IgG-Like bsAb: In a hybrid IgG-like/non–IgG-like bsAb, a traditional IgG half Ab is paired with an Fc-fused, non–IgG-like half Ab. One example under development by Teneobio pairs a fixed LC IgG half Ab with a VH fused directly to hinge–CH2–CH3 to form a half Ab. KiH modification to the two CH3 domains promotes heterodimerization (28).
Looking Ahead
In the upcoming second half of this review (BPI’s January–February 2018 issue), we will compare the features of IgG-like bsAbs with those of monoclonal antibodies and discuss therapeutic and diagnostic uses before addressing upstream production and downstream processing issues related to manufacturing IgG-like bsAbs.
Acknowledgment
We are grateful to Laura Shih for her contribution in preparation of the figures presented in this review.
References
1 Janeway CA Jr., et al. Immunobiology: The Immune System in Health and Disease, 5th Edition. Garland Science: New York, NY, 2001.
2 Linke R, Klein A, Seimetz D. Catumaxomab: Clinical Development and Future Directions. MAbs 2(2) 2010: 129–136.
3 Mullard A. FDA Approves First Bispecific. Nat. Rev. Drug Discov. 14(7) 2015; doi:10.1038/nrd4531.
4 National Cancer Institute. Blinatumomab. US National Institutes of Health: Rockville, MD, 2017; www.cancer.gov/about-cancer/treatment/drugs/blinatumomab.
5 National Library of Medicine. ClinicalTrials.gov. US National Institutes of Health: Rockville, MD; www.clinicaltrials.gov.
6 Chelius D, et al. Structural and Functional Characterization of the Trifunctional Antibody Catumaxomab. MAbs 2(3) 2010: 309–319.
7 Haense N, et al. A Phase I Trial of the Trifunctional Anti Her2 × Anti CD3 Antibody Ertumaxomab in Patients with Advanced Solid Tumors. BMC Cancer 16(7) 2016: 420–429; doi: 10.1186/s12885-016-2449-0.
8 Jäger M, et al. The Trifunctional Antibody Ertumaxomab Destroys Tumor Cells That Express Low Levels of Human Epidermal Growth Factor Receptor 2. Cancer Res. 69(10) 2009: 4270–4276; doi:10.1158/0008-5472. CAN-08-2861.
9 Fischer N, et al. Exploiting Light Chains for the Scalable Generation and Platform Purification of Native Human Bispecific IgG. Nat. Commun. 6, 2015: 6113–6124; doi:10.1038/ncomms7113.
10 Atwell S, et al. Stable Heterodimers from Remodeling the Domain Interface of a Homodimer Using a Phage Display Library. J. Mol. Biol. 270(1) 1997: 26–35.
11 Ridgway JB, Presta LG, Carter P. “Knobs-into-Holes” Engineering of Antibody CH3 Domains for Heavy Chain Heterodimerization. Prot. Eng. 9(7) 1996: 617–621.
12 Liu Z, et al. A Novel Antibody Engineering Strategy for Making Monovalent Bispecific Heterodimeric IgG Antibodies By Electrostatic Steering Mechanism. J. Biol. Chem. 290(12) 2015: 7535–7562; doi:10.1074/jbc.M114.620260.
13 Davis JH, et al. SEEDbodies: Fusion Proteins Based on Strand-Exchange Engineered Domain (SEED) CH3 Heterodimers in an Fc Analogue Platform for Asymmetric Binders or Immunofusions and Bispecific Antibodies. Prot. Eng. Des. Sel. 23(4) 2010: 195–202.
14 Labrijn AF, et al. Efficient Generation of Stable Bispecific IgG1 By Controlled Fab-Arm Exchange. Proc. Natl. Acad. Sci. 110(13) 2013: 5145–5150.
15 Schaefer W, et al. Immunoglobulin Domain Crossover As a Generic Approach for the Production of Bispecific IgG Antibodies. Proc. Natl. Acad. Sci. 108(27) 2011: 11187–11192.
16 Kienast Y, et al. Ang-2-VEGF-A CrossMab, a Novel Bispecific Human IgG1 Antibody Blocking VEGF-A and Ang-2 Functions Simultaneously, Mediates Potent Antitumor, Antiangiogenic, and Antimetastatic Efficacy. Clin. Cancer Res. 19(24) 2013: 6730–6740; doi:10.1158/1078-0432.CCR-13-0081.
17 Wranik BJ, et al. LUZ-Y, a Novel Platform for the Mammalian Cell Production of Full-Length IgG-Bispecific Antibodies. J. Biol. Chem. 287(52) 2012: 43331–43339; doi:10.1074/jbc.M112.397869.
18 Kostelny SA, Cole MS, Tso JY. Formation of a Bispecific Antibody By the Use of Leucine Zippers. J. Immunol. 148(5) 1992:1547–1553.
19 Bostrom J, et al. Variants of the Antibody Herceptin That Interact with HER2 and VEGF at the Antigen Binding Site. Science 323(5921) 2009:1610–1614; doi:10.1126/science.1165480.
20 Tustian AD, et al. Development of Purification Processes for Fully Human Bispecific Antibodies Based upon Modification of Protein A Binding Avidity. MAbs 8(4) 2016:828–838; doi:10.1080/19420862.2016.1160192.
21 Mazor Y et al. Improving Target Cell Specificity Using a Novel Monovalent Bispecific IgG Design. MAbs 7(2) 2015:377–389; doi:10.1080/19420862.2015.1007816.
22 Lewis SM et al. Generation of Bispecific IgG Antibodies by Structure-Based Design of an Orthogonal Fab Interface. Nat. Biotechnol. 32(2) 2014:191–198; doi:10.1038/nbt.2797.
23 Canfield SM, Morrison SL. The Binding Affinity of Human IgG for Its High Affinity Fc Receptor Is Determined By Multiple Amino Acids in the CH2 Domain and Is Modulated By the Hinge Region. J. Exp. Med. 173(6) 199: 1483–1491.
24 Li T, et al. Modulating IgG Effector Function By Fc Glycan Engineering. Proc. Natl. Acad. Sci. 114(13) 2017: 3485–3490; doi:10.1073/pnas.1702173114.
25 Coloma MJ, Morrison SL. Design and Production of Novel Tetravalent Bispecific Antibodies. Nat. Biotechnol. 15(2) 2011:159–163; doi:10.1038/nbt0297-159.
26 Doppalapudi VR et al. Chemical Generation of Bispecific Antibodies. Proc. Natl. Acad. Sci. 107(52) 2010: 22611–22616.
27 Rossi EA, Goldenberg DM, Chang CH. The Dock-and-Lock Method Combines Recombinant Engineering with Site-Specific Covalent Conjugation to Generate Multifunctional Structures. Bioconjug Chem. 23(3) 2012:309–323; doi:10.1021/bc2004999.
28 Buelow B et al. Development of a Fully Human T Cell Engaging Bispecific Antibody for the Treatment of Multiple Myeloma (poster); www.teneobio.com/development-of-a-fully-human-t-cell-engaging-bispecific-antibody-for-the-treatment-of-multiple-myeloma-poster.
Jennifer Bratt and Angela Linderholm are consultants, Bryan Monroe is senior consultant, and Steven Chamow is principal consultant at Chamow and Associates, Inc. in San Mateo, CA 94403; 1-650-345-1878; steve@chamowassociates.com; www.chamowassociates.com.