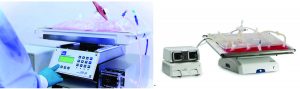
Figure 1: The original Wave Biotechnology rocking bioreactor (left) and Cytiva’s ReadyToProcess WAVE 20 bioreactor (right)
Over the past 10 years, a number of developments in disposable (limited use) and single-use technologies (SUTs) have been made for different bioprocess operations. Until recent years, much of the industry’s process equipment was sterilized using thermal methods such as autoclaving. Most equipment was reusable and required cleaning and sterilization before use. Such processes required validation and expensive and time-consuming resources. Production facilities relied on hard-piped, inflexible equipment such as large stainless-steel bioreactors and holding tanks. However, advanced SUTs now enable fast turnaround between batches and products, with rapid setup, increased flexibility, and fewer resources (compared with stainless-steel systems) required for cleaning, steaming, and cleaning validation. As a result, capital cost is reduced while speed to market authorization is increased.
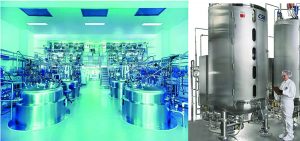
Figure 2: The head plates of traditional 20,000-L stainless-steel bioreactors (left) and ABEC’s CSR bioreactor (right)
Many single-use systems (SUS) are available for upstream processes such as cell-culture seeding, media preparation, aseptic sampling, aseptic connections/disconnections, and depth filtration. The number of disposable bioprocess applications is increasing, and industrial suppliers of SUSs continue to offer opportunities for reducing contamination risks and saving time.
Even though stainless-steel operations remain critical to the industry and continue to dominate commercial manufacturing, SUTs represent a new paradigm in the evolution of bioprocessing technologies.
The discussion herein focuses on advances in disposable and single-use scale-up, single-use bioreactors and cell-culture seeding operations, and other operations with disposable components such as aseptic sampling, tubing, filtration, and aseptic connection. The advantages and challenges of disposables and SUTs compared with those of stainless-steel systems in cell-culture processing also are explored. Other processing areas are outside the scope of this review.
Demand for SUTs
The increased demand for biopharmaceuticals continues to drive investment in new manufacturing facilities. Some of those are starting to look rather different from traditional sites based mainly on stainless steel (1). Large-scale facilities traditionally restricted the use of SUTs (e.g., shake flasks) to early inoculum stages of cell culture. Now, implementation of single-use/disposable technologies is expanding to all areas of biopharmaceutical production — from early inoculum stages to final filling. In September 2017, Eurofins Amatsigroup’s “Disposable-Lab” facility was the first to manufacture an injectable clinical batch within a single-use flexible film isolator (which was developed by Solo Containment) (2).
A number of factors have contributed to the increasing use of SUTs in biomanufacturing. These factors include a need to lower capital costs, shorten change-over times between batches, increase process flexibility, and reduce risk of crosscontamination between batches (3). When implementing SUTs, no clean-in place and steam-in-place (CIP/SIP) operations are required because disposable technologies generally are sterilized with gamma radiation. Thus, SUT piping and instrumentation are significantly simpler than those for traditional stainless-steel equipment (4).
Upstream Applications
Disposable Bioreactors: Over 60% of typical stainless-steel bioreactor instrumentation is designed for CIP and SIP operations (4). Advances in production technologies have led to higher product titers. Consequently, bioreactor scales have decreased. Today, one 2,000-L single-use bioreactor can produce the same titers that a 20,000-L stainless-steel bioreactor could accomplish just a few years ago (1, 5).
Rocking Motion: The first single-use bioreactor was launched in 1996 by Wave Biotechnology (5). It consisted of a pillow-shaped disposable bag that inflated to sustain a rigid structure. The bag was placed on a heated platform. No mechanical agitators were required in the bag. Agitation was achieved by rocking the platform at set frequencies. But product titers with this type of reactor were not adequate to meet large-scale capacity needs at that time, so the use of Wave bioreactors was limited. Currently, the biopharmaceutical industry’s needs have shifted to large-scale manufacturing and the use of rocking-motion bioreactors has increased. In 2007, GE Healthcare (now Cytiva) acquired Wave Biotechnology (6). Today, Cytiva’s ReadyToProcess WAVE 25 rocker bioreactor is similar in design to the original Wave bioreactor launched over two decades ago (Figure 1).
In 2004, the first single-use stirred tank bioreactor was launched by HyClone (now part of Thermo Fisher Scientific) with a 250-L capacity (3, 7). Agitation was achieved by a built in single-use impeller that was integrated into the bag design. When the bag was mounted, the impeller was magnetically connected to a driver shaft. In 2009, the first 2,000-L single-use bioreactor was launched. In In September 2019, ABEC set a new benchmark and announced the release of its 6,000-L capacity custom single run (CSR) single-use bioreactor (Figure 2), currently the largest single-use bioreactor available on the market (8).
Other single-use bioreactor designs are offered by Merck Millipore, Pall Life Sciences, Applicon, and Thermo Fisher Scientific. Even though many designs are available, they all include similar features such as sampling and monitoring ports as well as aseptic liquid addition and transfer capabilities. They also feature temperature sensing, gas venting, and air sparging — all common features found in traditional stainless-steel bioreactors as well.
Several small-scale benchtop single-use bioreactors are on the market. Figure 3 shows a 2-L UniVessel single-use bioreactor by Sartorius Stedim, made available in 2011 (9).
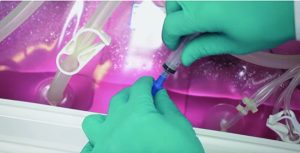
Figure 4: A syringe connected to a Clave Luer lock for aseptic sampling of a rocking-motion bioreactor
Sampling Systems and Devices: Frequent aseptic sampling of a bioreactor culture is required to maintain and confirm that conditions in the vessel are as desired (10, 11). Singe-use bioreactors generally are sampled through ports equipped with a Clave Luer lock (from ICU Medical) connection such as that found on the bioreactor bag in Figure 4. A needle-free syringe is connected to the Luer lock by an operator pushing it in and twisting it in place. Such connections have been validated for up to 50 samples according to Sartorius Stedim.
Sampling from stainless-steel bioreactors with disposable technologies generally is performed using sterile sampling devices such as a NovaSeptum sampling device (from Merck/MilliporeSigma) (Figure 5). Sampling bags are loaded onto a NovaSeptum holder and locked into place on a bioreactor port before performing a standard SIP on the bioreactor. To begin sampling, an operator presses the NovaSeptum trigger, causing the sampling needle to pierce the silicone diaphragm aseptically so that a sample to be taken from the bioreactor without compromising sterility. When enough sample is taken, the operator releases that trigger and places it in the “lock” position. The sampling bag is crimped manually using the crimping tool, thus aseptically separating the sampling bag from the NovaSeptum system connected to the bioreactor (12, 13). The NovaSeptum system also can be connected to a stainless-steel vessel sampling port for sampling in a similar way. Sample volumes can be as small as 1 mL using syringes and up to 1,000 mL using sampling bottles.
Other similar sampling systems include the Gore Sta-Pure fluid-sampling system and AllPure’s (acquired by Sartorius Stedim in 2014) TakeOne sampling system (14). Both are fully disposable, including their holders. By contrast, the NoveSeptum sampling system has stainless-steel holders that must be cleaned after use.
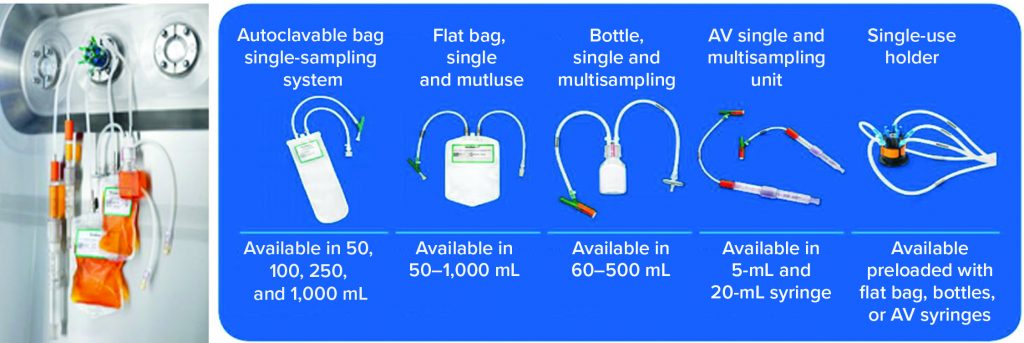
Figure 5: Merck’s NovaSeptum sampling system connected to a stainless-steel bioreactor port (left) and NovaSeptum container designs for sampling (right)
Connections and Disconnections: Typically, when operators transfer aseptic fluid from one vessel/bioreactor to another, they use tube welders. After the transfer is complete, tube sealers are used to disconnect both systems from each other aseptically. Disposable technologies such as aseptic connections and disconnections are alternatives to tube welders and sealers (15).
For example, aseptic transfer of media from a bag into a bioreactor (disposable or stainless steel) requires that a line of tubing on both bag and bioreactor is preaffixed to a connector end that has a removable membrane or valve barrier for mating to another connector end (16). Several connector designs are available, both genderless and traditional male–female components (Table 1).
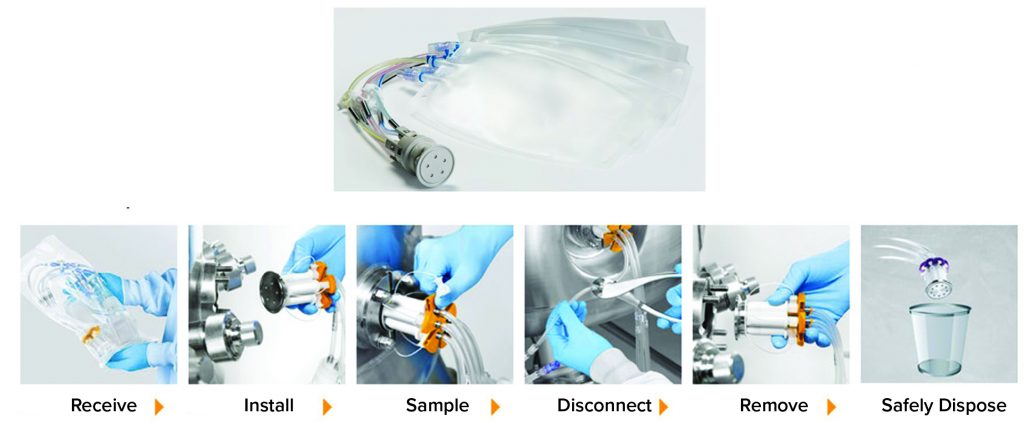
Figure 6: Gore’s Sta-Pure fluid sampling system (top) and the TakeOne assembly and disassembly procedure (bottom)
Connectors can be supplied by a manufacturer as part of a gamma irradiated disposable media bag or can be bought separately, attached to assemblies in-house, and then autoclaved for sterility. Traditionally, a connector would work only with a bag from the same manufacturer (e.g., a Sartorius connector could be connected only to Sartorius disposable media bags). But now, suppliers have become more flexible, and you can buy a Pall bag with a Sartorius connector attached to it.
Suppliers such as Colder Products Company (CPC), Pall, and Sartorius Stedim offer aseptic disconnectors as alternatives to tube sealers (Figure 7). The first two in the figure can be preaffixed to tubing on a bag manifold, and the third operates in a different way (described below). The CPC AseptiQuik DC disconnector is a two-in-one connector and disconnector.
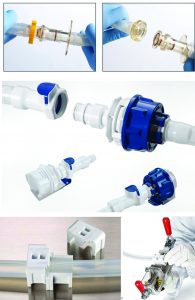
Figure 7: Pall Kleenpak disconnector before and after disconnection (top), CPC AseptiQuik DC sterile connector and disconnector (middle), and Sartorious Stedim Clipster aseptic disconnector and handheld cutting tool (bottom)
The Clipster aseptic disconnector from Sartorius Stedim is used after fluid transfer is complete. It can be preassembled as part of a fluid-transfer bag, for example, or it can be assembled onto tubing and disconnected when needed. After the assembly is complete, the tube cutter is used to cut tubing aseptically. Then the disconnector is removed. The aseptic disconnector comes in different sizes based on thickness of the tubing to be cut. The company also offers an alternative, preinstalled disconnection method (QuickSeal disconnect) that basically is a metal collar. With it, tubing is aseptically disconnected with a crimper.
Filters: Filtration is a fundamental part of all bioprocesses, from upstream to sterile filtration of a drug product before fill–finish steps (17). A range of filter types and sizes are used in different areas. For example, in upstream processing suites, both hydrophobic and hydrophilic filters can be used. Hydrophobic filters act as vent filters for both stainless-steel and single-use bioreactors, stainless-steel vessels, and disposable bags. All these filters come in different pore sizes, with the most common being 0.45-µm (bioburden-reduction filter) and 0.2-µm (sterilizing-grade filter).
Filters used with stainless-steel systems typically are contained in stainless-steel housings during use. Those associated with SUTs are fully disposable and housed in disposable plastics (Figure 8). Example filter types include the Sartofluor range (from Sartorius Stedim), the Advanta range (from Pall), and the ULTA Prime and ULTA Pure ranges (from Cytiva).
Other filter types used in upstream processing include microfiltration cassettes for cell harvesting, and disposable depth filters — also referred to as Pod filters (from Millipore) — used in a polishing step after harvesting (18, 19).
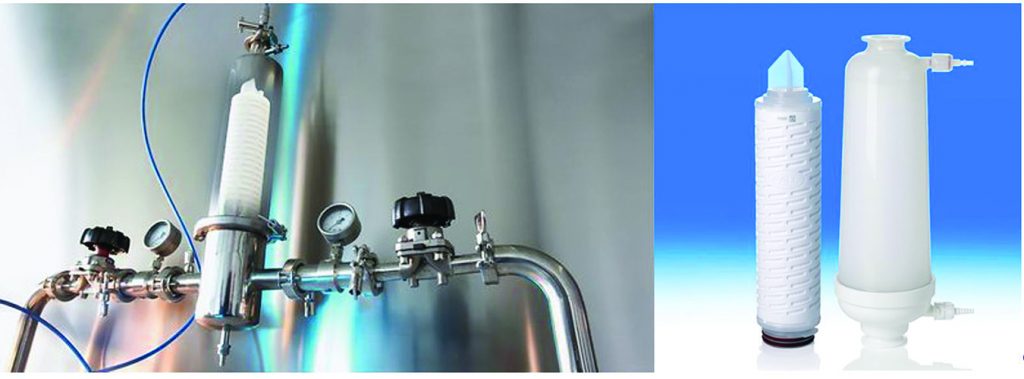
Figure 8: A gas vent filter assembled on a stainless steel skid to be used with a stainless steel housing (left); a disposable filter housed in a plastic white capsule (right)
Sensors: Production processes must be monitored to determine whether all critical process parameters (CPPs) are maintained within specifications. Examples include the monitoring of pH and dissolved oxygen (DO) during cell culturing in a bioreactor.
Different types of sensors are used either on-line or off-line to measure CPPs. On-line sensors can be in-line or at-line. In-line sensors interface directly to a bioreactor and are in contact with contents in it. At-line sensors are used when a sample is taken and analyzed outside a bioreactor but in close proximity to it. Off-line samples are taken from a bioreactor and analyzed in a laboratory area away from the bioreactor (20, 21).
Process-monitoring sensors and probes can be connected to disposable bioreactors. Some of these sensors enable real-time monitoring, aligning well with the industry’s move toward implementing process analytical technology (PAT) systems (22). Such technologies can be categorized as follows.
Preinstalled pH and DO patches are connected to noninvasive fiber-optic cables (reusable cables). These devices rely on fluorescent-based detection of pH and DO (e.g., Sartorius disposable bioreactors operate using such probes).
Traditional probes are attached to an aseptic connector (e.g., Pall Kleenpak in Figure 9). Thermo Scientific and Cytiva offer similar setups (23).
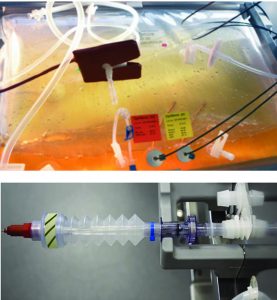
Figure 9: Rocking-motion bioreactor with noninvasive optic sensors attached (top) and Pall’s Kleenpak bellow assembly (bottom)
Optical sensors for measuring pH and DO are easy to use and set up. However, their measurements can be inaccurate and tend to drift. Traditional methods of measuring pH and DO using preautoclaved probe assemblies aseptically inserted into ports are more reliable (24). That might be why most bioreactor manufacturers offer those types of ports on their single-use bioreactors even though insertion of the probes is much riskier than using noninvasive types. Suppliers such as Sartorius Stedim and Pall offer preinstalled, single-use pH probes as part of disposable mixing-bag systems.
Benefits and Challenges
To eliminate the need for laborious cleaning of product-contact equipment, disposables initially were used in biomanufacturing processes as alternatives to reusable consumables and equipment. Doing so also eliminated required cleaning validation, reduced risk of crosscontamination, and improved sterility assurance (22).
SUTs have reduced dependency on utilities for sterilizing product-contact parts of bioreactors between batches. Thus, processing times have been shortened, operational costs have been lowered, and cleaning validation and training have decreased (25). The environmental impact brought on by disposing of SUTs has been studied and determined to be offset by reduced dependencies on energy and disposal of cleaning materials. SUTs also provide process flexibility, thus decreasing the time needed to bring therapies to market (4).
But disposable technologies still have limited scalability compared with other options. They also lack of industry-wide standardization between vendors, so biomanufacturers greatly depend on suppliers. SUT end users take on the cost of waste disposal and must address risks associated with leachables and extractables, which can contaminate drug-product solutions and inhibit cell growth in a bioreactors (16). Table 2 lists some advantages and drawbacks of using SUS.
Meeting Market Demands
The global need for high-quality biopharmaceuticals continues to encourage manufacturers to reduce costs and shorten times to market. By implementing disposable technologies, innovating new SUS, and updating processing equipment, drug developers can address market demand, ultimately benefiting patients.
Initially, disposable technologies were designed as alternatives to reusable consumables and equipment as a means of eliminating tedious cleaning processes.
Today, they are resilient competitors to traditional biomanufacturing equipment and essential parts of bioprocessing. Several start-up companies have built their facilities to include mostly SUTs. Examples include WuXi Biologics in Ireland, which is building the industry’s largest single-use bioreactors (26), and Shire (now Takeda) has adapted SUTs in its new facility in Dunboyne, Ireland (27).
Disposable technologies offer many advantages but still have many limitations such as those discussed above. However, it is worth noting that some manufacturers continue to favor traditional stainless-steel systems due to their proven performance.
References
1 Tiene G. Single-Use Now Key Technology in Biopharmaceutical Manufacturing. Pharm. Outsourc. 31 January 2016.
2 Amatsigroup Manufactures World’s First Injectable Clinical Batch Under Single-Use Isolator. Contract Pharma 5 September 2017.
3 Shukla A, Gottschalk U. Single-Use Disposable Technologies for Biopharmaceutical Manufacturing. Trends Biotechnol. 31(3) 2013: 147–154; https//doi.org/10.1016/j.tibtech.2012.10.004.
4 Markarian J. Single-Use Technologies Transform Biopharmaceutical Facilities and Manufacturing Equipment. Pharm. Technol. 10(8) 2017; http://www.pharmtech.com/single-use-technologies-transform-biopharmaceutical-facilities-and-manufacturing-equipment.
5 Subramanian G. Continuous Biomanufacturing: Innovative Technologies and Methods. Wiley VCH Verlag GmbH & Co. KGaA: Weinheim, Germany, 2017: 151–152.
6 Van Arnum P. GE Healthcare Acquires Wave Biotech. Pharm. Technol. 19 April 2007; http://www.pharmtech.com/ge-healthcare-acquires-wave-biotech.
7 Kinsella JK, Dewan SS. Single-Use Market: Rise of Single-Use Technologies and Systems in Biopharmaceuticals. Drug Dev. Deliv. 15(9) 2015; https://drug-dev.com/single-use-market-rise-of-single-use-technologies-systems-in-biopharmaceuticals.
8 Barba V. ABEC Launches 6,000-L Single-Use Bioreactor to Answer Scalability Needs. BioPharma Reporter 12 September 2019; https://www.biopharma-reporter.com/Article/2019/09/12/ABEC-launches-6-000L-single-use-bioreactor.
9 Sartorius Stedim Biotech Launches UniVessel SU Single-Use Bioreactor for Small-Scale Cell Culture Applications. B3C Newswire 9 May 2011; https://www.b3cnewswire.com/20110509495/sartorius-stedim-biotech-launches-univessel-su-single-use-bioreactor-for-small-scale-cell-culture-applications.html.
10 Trotter M. Bioreactor Sampling. Manufacturing.Net 25 March 2013; https://www.manufacturing.net/energy/article/13150730/bioreactor-sampling.
11 Challener C. Advances in Real-Time Bioreactor Sampling. Pharm. Technol. 2014.
12 Clark KJR, Furey J. Suitability of Selected Single-Use Process Monitoring and Control Technology. BioProcess Int. 4(4) 2006: S16–S20.
13 NovaSeptum Sampling Systems. EMD Millipore 2012.
14 Stanton D. Sartorius Acquired AllPure to Fill Gaps in Single-Use Portfolio. Biopharma Reporter 29 April 2014; https://www.biopharma-reporter.com/Article/2014/04/29/Sartorius-acquires-AllPure-to-fill-gaps-in-single-use-portfolio.
15 Mach CJ, Riedman D. Reducing Microbial Contamination Risk in Biotherapeutic Manufacturing. BioProcess Int. 6(8) 2008: 20–26; http://lne.e92.mwp.accessdomain.com/analytical/upstream-validation/reducing-microbial-contamination-risk-in-biotherapeutic-manufacturing-183152.
16 Harper K, Strahlendorf KA. A Review of Sterile Connectors. BioPharm Int. 2 November 2009; https://www.biopharminternational.com/review-sterile-connectors.
17 Van Reis R, Zydney A. Membrane Separations in Biotechnology. Curr. Op. Biotechnol. 12(2) 2001: 208–211; https://www.ncbi.nlm.nih.gov/pubmed/11287239.
18 Stock RA. Significant and Growing Market: Single-Use Technology in the Biopharmaceutical Industry. Am. Pharm. Rev. 1 October 2010; http://www.americanpharmaceuticalreview.com/Featured-Articles/115054-A-Significant-and-Growing-Market-Single-Use-Technology-in-the-Biopharmaceutical-Industry.
19 Schmidt SR, Wieschalka S, Wagner R. Single-Use Depth Filters: Application in Clarifying Industrial Cell Cultures. BioProcess Int. 15(1) 2017: 18–24; http://lne.e92.mwp.accessdomain.com/2017/single-use-depth-filters-application-clarifying-industrial-cell-cultures.
20 Lourenco ND, et al. Bioreactor Monitoring with Spectroscopy and Chemometrics: A Review. Anal. Bioanal. Chem. 404(4) 2012: 1211–1237; https://doi.org/10.1007/s00216-012-6073-9.
21 Busse C, et al. Sensors for Disposable Bioreactors. Engin. Life Sci. 18(8) 2017: 940–952; https://doi.org/10.1002/elsc.201700049.
22 Sandle T. Strategy for the Adoption of Single-Use Technology. Eur. Pharm. Rev. 23(1) 2018: 43–45; https://www.europeanpharmaceuticalreview.com/article/73939/strategy-for-the-adoption-of-single-use-technology.
23 DePalma A. See the Bioprocess, Be the Bioprocess. Gen. Eng. Bioprocess. News. 36(15) 2016; https://www.liebertpub.com/doi/full/10.1089/gen.36.15.12?src=recsys.
24 Zhou W, Kantardjieff A. Mammalian Cell Cultures for Biologics Manufacturing. Springer: Berlin, Germany, 2014.
25 Gottschalk U. Disposables in Downstream Processing. Adv. Biochem. Eng. Biotechnol. 115, 2010: 171–183; https://doi.org/10.1007/10_2008_22.
26 WuXi Biologics to Invest €325 Million to Build Largest Biomanufacturing Facility Using Single-Use Bioreactors in Ireland. WuXi Biologics, 30 April 2018; https://www.wuxibiologics.com/wuxi-biologics-invest-e325-million-build-largest-biomanufacturing-facility-using-single-use-bioreactors-ireland.
27 Shire Announces Collaboration with National Institute of Bioprocessing Research and Training: Shire to Utilise NIBRT-GE’s Single-Use Centre of Excellence. Meath County Council, 2018; https://www.manufacturingchemist.com/news/article_page/Shire_announces_collaboration_with_National_Institute_of_Bioprocessing/141185.
Shada Warreth is senior bioprocessing trainer and training coordinator at the National Institute for Bioprocessing Research and Training (NIBRT), Foster Avenue, Mount Merrion, Blackrock, Co. Dublin, Ireland; shada.warreth@nibrt.ie.