Most commercial biopharmaceuticals originated from academic research laboratories and start-up development laboratories. Despite such products having differences in modalities and targeted disease indications, and whether their target patient populations are relatively small or approaching blockbuster status, at a key point in development, biopharmaceutical production must scale up from laboratory to commercial production. That movement from research to development and then to manufacturing forces attention on economics and speed to market, and it drives innovative approaches to producing biopharmaceutical cell compositions at commercially relevant scales and at cost structures that support business models.
Regenerative medicines, whether for cell therapies or biofabricated tissues, now face that same transition. Bioprocess developers require a steady supply of consistent, highly functional, highly viable cells at large volumes for both product and process developing and clinical manufacturing. Stem cells and other primary cells, most of them adherent in nature, have a strong history as tools for scientific research and for learning about mechanistic biology. However, primary cells have now graduated from a simple research tool to the class of a true “technology.†Over the past couple decades, the biopharmaceutical industry has gained a greater understanding of aspects around primary cell manufacturing, scale-up, quality parameters, in-process controls, and the regulatory sciences for this broad class of products. That has laid a foundation for an acceleration in the adoption of cellular technologies into regenerative medicine products. Therapeutic adherent cells have taken the same path as the technological components of electronic devices — transistors and microchips, for example. Adherent cells are raw materials needed by regenerative medicine product developers who are creating emerging therapies in simple-to-use (or “plug and playâ€) formats.
That philosophy has laid the foundation for innovative companies such as RoosterBio. The goal of the company is to deliver adherent cells in formats that enable consistent, simple, and cost-effective incorporation into products, thereby reducing the time an innovator has to spend during all stages of product development, technology transfer, and clinical manufacturing. Those cellular technologies must be developed to consistent quality specifications and delivered to product developers and manufactures for use.
RoosterBio was launched with the realization that the human mesenchymal stem/stromal cell (hMSC) field needed tools to simplify manufacturing cells in large volumes and with scalable processes. Five years ago, the company began by offering ready-to-use hMSC bioprocess systems in the form of cell banks and highly engineered culture media. They were designed to be “off-the-shelf†products, with standardized quality and standardized protocols for working with them so that anybody with basic cell culture skills could become an expert stem cell manufacturer by acquiring the cell bank and media systems. An innovator can simply and quickly generate significant volumes of hMSCs to perform product development and enter clinical translation rapidly — cutting years from typical regenerative medicine development time lines. Newly formed product teams within industry with no previous hMSC experience, for example, can access sophisticated hMSC components to develop new product concepts. If successful, such products have a scalable raw material (the hMSC) manufacturing process “built in.â€
Advancing Manufacturing Sciences
Similar to all technological industries, cell-based products have followed the product cycle of innovation (Figure 1) (1). First, there is a wave of product innovations followed by a wave of manufacturing process innovations. The regenerative medicine field currently is at an inflection point of maximal rate of manufacturing process innovation (arrow in Figure 1). For cell therapies, that process might be first making T cells and then gene-edited TÂ cells or first making induced pluripotent stem cells (iPSCs) and then gene-edited iPSCs and MSCs.
As products approach commercialization, manufacturers need to make products at commercially relevant scales and with cost structures that will support a business model. The bioindustry is entering a stage of massive bioprocess innovation at which a single cell type can be used for many different therapeutic indications. That advancement is being supported by organizations such as the National Institute for Innovation in Manufacturing Biopharmaceuticals (NIIMBL) and BioFabUSA, part of the Advanced Regenerative Manufacturing Institute (ARMI). Such government-funded agencies are investing in manufacturing sciences specifically for cellular medical technologies, paving the way for rapid developments in the fields of biofabrication and regenerative medicine manufacturing.
As adherent cells, hMSCs are critical components for tomorrow’s cell and gene therapies, engineered tissues, and combination medical devices. Thus, economical production systems must be developed. That requires engineering media systems for productivity and efficiency because culture medium always is a major cost driver of manufacturing. Hyper-efficient medium creates a foundation for lower cost components of regenerative medicine products. Standardization of manufacturing processes and starting materials also bring newfound efficiencies to living cell technologies. The end goal is to create mass availability of what traditionally has been a scarce resource (cells) available only to the best-funded companies or laboratories.
Such factors are driving not only the transition from flasks into multilayer vessels and then into bioreactor production platforms, but also the sophisticated engineering of media systems specifically designed to enhance cellular productivity. The media productivity metric that is analogous to grams per liter used in the monoclonal antibody (MAb) space is millions of cells produced per liter of media consumed. Until recently, that metric was not promoted for cell therapy manufacturing, but it is required to move the field toward a commercial mindset.
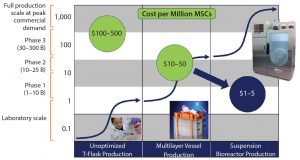
Figure 2: Manufacturing platform evolution will drive the economics of stem cell supply for years to come (3, 4).
Figure 2 compares hMSC productivity across different two- and three-dimensional systems. Taking a “bioprocess approach†toward adherent cell production is helping manufacturers take important steps toward standardization in regenerative medicine.
MSCs as Foundational Technologies
Human MSCs are used widely as components for the clinical development of regenerative medicine products, with applications ranging from immune modulation to engineered tissues and organs. These cells have been used in more than 30,000 patients to date through registered clinical trials and perhaps an additional tens of thousands of stem cell “tourists.†hMSCs have had a good safety profile, but the current focus of attention is on maintaining or improving functionality through manufacturing sciences.
New gene-editing technologies are enabling scientists to edit MSCs and other cell types to increase cell potency. The research fields of extracellular vesicles and exosomes also need significant numbers of hMSCs. Cell numbers represent a considerable bottleneck in biofabrication research and product development — slowing the pace of innovation in that field. But bioprocess innovations are poised to end that supply constraint. Tens of product companies today soon will expand to hundreds of companies that all need a steady supply of high-quality hMSCs and scalable production technologies. Those companies will need to obtain billions of cells for development and then hundreds of billions of cells to support commercial-scale manufacturing.
A Technology Roadmap
The National Cell Manufacturing Consortium (a NIST-driven cell manufacturing group, for which the Georgia Institute of Technology took the leadership role) has developed an upstream and downstream technology roadmap (2). Figure 2 shows the evolution of manufacturing platforms from multilayer vessels to bioreactors, a process that will drive the economics of adherent-cell supply for years to come. Lot size (y axis) is on a log scale, and the x axis shows the technology platform. The technology S-curves in Figure 2 show where adherent cell production constrains are for each platform (3–5). Each technology production platform usually is good for a log or two of productivity, after which you move to the next technology platform to reach desired lot sizes.
For hMSCs, and other adherent cells, production in T-flasks easily can make 5–100 million cells. But with this platform, generating a larger number of cells is much more difficult if your need approaches a billion cells. The next platform is a multilayer vessel system that can produce 5–15 billion cells easily, but tens of billions would be difficult. At that point, suspension bioreactors are required to achieve lots of 50–100 billion cells. Although a small-scale bioreactor can produce a billion cells, using multilayer vessels is more efficient at that scale. For producing 50 billion cells or more, multilayer vessels are no longer feasible. Single-use suspension bioreactors currently are enabling scale-up to hundreds of billions of cells, likely topping out at about a trillion cells (4).
Economics of Cell Production
The development of efficient, scalable technologies for producing large volumes of cells is driving bioprocess economics. The main bioprocess economic metric that cell therapy manufacturers have been following for the past several years (at least for MSCs) has been the “cost per million cells.†That varies according to cell type. For MSCs, cost varies with lot size and process engineering. Depending on scale and level of streamlining, the cost per million hMSCs produced can range from hundreds of dollars per million cells when lot sizes are in the tens of millions, to tens of dollars when lot sizes are in the low billions. Costs of <$10 per million hMSCs can be achieved as lot sizes surpass 100 billion cells/lot.
The impact of lot size and process engineering on hMSC economics is consistent with the microprocessor field’s Moore’s law — just applied to stem cells. Moore’s law can be applied to nearly any technology, including biotechnologies and directly to adherent cells (6). Like many new technologies, stem cells will continue to decline in cost.
Reducing the cost of adherent cells to tens of cents per million cells will require future technology innovation step changes, but RoosterBio experts expect to see those changes happening within 10–15 years. The industry is poised to deliver hMSCs in the range of $1–5 per million cells (the next log-reduction level), but the demand for those cells must be sufficient to support that lot size. As the first hMSC products achieve blockbuster status (>$1 billion in revenue, likely treating >100,000 patients per year), the economies of scale will drive their costs to single-digit dollars per million cells. So achieving such efficiencies is both technology and demand driven.
RoosterBio was founded specifically to drive rapid adoption of producing MSCs in large volumes with scalable processes that will be consistent with favorable economics. Making 100 million cells typically takes well over a month and incurs expenses for cell culture media that drive up the cost of goods. The company’s approach is to provide one bottle of media that in five days could be expanded to 100 million high-quality MSCs, and with further passaging could be expanded to more than a billion cells.
Rapidly achieving a billion cells allows a company to develop and innovate a number of products rapidly, thus accelerating the field of hMSC-based products. With platforms that can generate cells quickly (in millions produced per liter of media consumed), the industry can start discussing productivity metrics for regenerative medicine the way it does for biotherapeutics (in g/L). That approach enables 10× productivity enhancements in much shorter time frames than those mentioned above.
A Novel Strategy
With a closed-system platform that includes xeno-free (XF) cell culture media and XF cells, RoosterBio laid the groundwork for its current good manufacturing practice (CGMP) products launched earlier this year. Since then, the company has collaborated with PBS Biotech to help therapeutics companies and manufacturers get up and running in bioreactor-based suspension culture systems.
RoosterBio has been granted funding through the Department of Defense (DoD)-funded Medical Technology Enterprise Consortium (MTEC), in collaboration with BioBridge Global, GenCure, and the United States Army Institute of Surgical Research to establish a 50-L XF MSC manufacturing process. That effort consists of developing a seed train and a suspension culture process that scales up to an 80-L PBS bioreactor, a scale that is critical to generate the cell numbers necessary for clinical therapies. The process incorporates XF cells, XF microcarriers, and an XF fed-batch bioprocess media system. The downstream process will include microcarrier separation, continuous centrifugation for volume reduction and cell washing, vial filling, controlled rate cryopreservation, and storage, with each unit operation platform identified by the process scale.
The company is developing this process and transferring the technology to BioBridge Global’s GenCure business unit. The goal is to standardize the bulk cell expansion of the process, which can be used by any company to grow a vast quantity of MSCs. The hMSCs then can be used by therapeutic developers for different downstream applications, including exosome production, bioprinting of engineered tissues and organs, or gene-edited cell therapies. Having a standardized hMSC production platform for further engineering, the company is hoping to achieve an impact similar to what Lonza experienced with its GS-CHO cell line for biotherapeutics. When that cell line came to the market, Lonza scientists knew how to scale it quickly to 10,000–20,000 L bioreactors to make a wide variety of proteins – bringing newfound efficiencies to biotherapeutic product development.
RoosterBio is taking a similar approach to hMSC production: a standardized CGMP manufacturing process that anyone can access for any application or for transfer to a CMO to reduce the time needed for process development. The company starts by showing that a production process is working correctly in a 0.1-L bioreactor and then scales up the process to a 3-L bioreactor system. The hMSC seed train ends on microcarriers expanded in XF media, then an XF bioreactor feed is added three days later, and the cells are harvested for applications after five or six days in culture. The process has been shown to work in multiple donors and is repeatable, with good comparability of quality attributes. Cell surface marker expression coming out of the bioreactor is consistent with that in flask expansion, and the cytokine secretion profile and immunodulatory functions of the MSCs between two dimensional culture and three dimensional culture also is comparable.
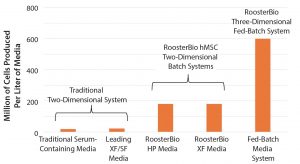
Figure 3: Fed-batch bioreactor expansion enhances hMSC media productivity (XF = xeno free, SF = serum free).
The transition from traditional media exchange flask culture to a batch flask culture and then to fed-batch bioreactor expansion demonstrates increasing media efficiency as measured by cells produced per liter of media consumed. Traditional two-dimensional (2D) cultures provide tens of millions of cells per liter, and batch 2D systems demonstrate enhanced productivity (Figure 3). Bioreactor expansion using fed-batch media systems are much more efficient in media use, tripling culture productivity. Because media is one of the most significant costs drivers, overall productions costs are lowered as a process moves to more efficient production platforms.
A Boost for Innovation in Clinical Manufacturing
Traditionally, if a company wanted to start clinical development with an adherent-cell therapy, it would need at least three years to develop and produce master and working cell banks from which production would begin. A standardized platform that can generate fully tested, ready-to-go CGMP cell banks can reduce product development costs and time for regenerative medicines and shorten time to clinical trials.
With such a platform, several companies can work off of one cell bank for early clinical testing, making the entire industry much more efficient and driving it toward improved bioprocess economics. Costs of $1–5 per million cells cannot be reached by making just 10 billion cells. Instead, such cost efficiencies come with producing hundreds of billions of cells. But the regenerative medicine industry is well on its way to increasing cost efficiencies, and platforms such as those by RoosterBio are laying the groundwork for this Moore’s law of stem cells. As demand for such products increases, the industry will have finally achieved truly cost-effective cellular therapies.
Considering the rate of innovation for an industry (Figure 1), as manufacturers determine solid product specifications and invest in the manufacturing sciences, they create the technology foundation for commercial supply of cost-effective regenerative medicines. RoosterBio believes that next-generation product innovation is going to increase dramatically when such new products can be developed at much lower costs than are currently possible.
Cell therapy innovations can follow the same path as other technologies. For example, cell phones have benefited from convergent innovations, including touch-screen technologies, GPS, and camera systems — all in one small package. Such advances all benefited from years of individual development from several different fields. That is essentially what companies such as RoosterBio are working toward with “standardized†platforms and dedicated teams of individuals who are focused on driving a new development of MSCs.
References
1 Abernathy WJ, Utterback JM. Patterns of Industrial Innovation. Technol. Review 80(7) 1978: 40–47.
2 Achieving Large-Scale, Cost-Effective, Reproducible Manufacturing of High-Quality Cells: A Technology Roadmap to 2025; February 2016; cellmanufacturingusa.org.
3 Simaria AS, et al. Allogeneic Cell Therapy Bioprocess Economics and Optimization: Single-Use Cell Expansion Technologies. Biotechnol. Bioeng. 111(1) 2014: 69–83; doi:10.1002/bit.25008.
4 Rowley J, et al. Meeting Lot-Size Challenges of Manufacturing Adherent Cells for Therapy. BioProcess Int. 10(3) 2012: 16–22.
5 Olsen TR, et al. Peak MSC — Are We There Yet? Front. Med. 21 June 2018; https://doi.org/10.3389/fmed.2018.00178.
6 Rob Carlson. Biology Is Technology: The Promise, Peril, and New Business of Engineering Life, Harvard University Press: Cambridge, MA, 2011.
Jon Rowley is founder and chief product officer at RoosterBio; jon@roosterbio.com. S.Anne Montgomery is editor in chief and cofounder of BioProcess International; amontgomery@bioprocessint.com.