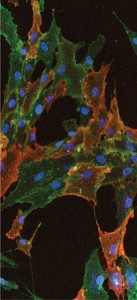
Immunocytochemical staining shows cultured human bone marrow–derived mesenchymal stem cells stained with STRO-1 and CD90 antibodies. Nuclei of the cells are
visualized using DAPI (blue).
EMD MILLIPORE
Cell therapy holds the promise of delivering the next generation of future medical breakthroughs. In this respect, multipotent progenitor cells such as human mesenchymal stem cells (hMSCs) have attracted high clinical interest because of their ability to differentiate into various cell types and their immunoregulatory properties. Furthermore, hMSCs express only low levels of class I major histocompatibility complex (MHC I) molecules on their surfaces and are therefore invisible to a host’s immune system. Finally, hMSCs can actively suppress the innate immune system by expressing a number of secreted factors such as factor H and human leukocyte antigen G5 (HLA-G5).
Together, those features enable the allogeneic use of hMSCs and thus make them an attractive target for commercial therapeutic development. Allogeneic therapies have the advantage over autologous therapies of the potential for centralized manufacturing, storage, and distribution similar to classical therapeutic agents such as small molecules and biologics. In addition, allogeneic cell therapies can be produced at large scales, which will be imperative to ensure commercial viability.
Currently, large-scale expansion of hMSCs is mostly performed using traditional flatbed cultures (e.g., in flasks) and multilayer vessels such as 10-layer and 40-layer stacked trays, enabling lot sizes of 10–50 billion cells (1). Although such lot sizes are sufficient to satisfy the needs of clinical development, it is widely believed that multilayer vessels will not constitute a cost-effective approach for marketed products. Thus, significant efforts are under way to develop alternatives to flatbed cultures.
One promising approach is the expansion of hMSCs on microcarriers in stirred-tank bioreactors (2–4). This technology will allow for development of scaled-up processes in which expansion can go from small-scale vessels (e.g., 3 L) to large vessels (e.g., 1,000 L). Although such processes are still in development, it can be estimated that a single 1,000-L bioreactor could provide enough cells to produce thousands of clinical hMSC doses. Thus, microcarrier-based expansion of hMSCs offers an attractive process for allogeneic cell therapy products.
In addition to the choice of platform technology, the composition of a cell culture medium used for hMSCs is of significant concern for development of allogeneic cell therapy products. To date, nearly all such products in clinical trials require added serum in their growth media for expansion, most prominently fetal bovine serum (FBS). But that poses an important risk because current good manufacturing practice (CGMP)–quality FBS is of limited availability; a single commercially successful cell therapy product could deplete global FBS supplies.
The risk of unknown infectious reagents in FBS is also a significant concern. And some studies of it have demonstrated a risk to patients of immunogenic reactions to xenogenic proteins, which could be transmitted to hMSCs from FBS during culture (5). Thus, alternatives to FBS are under investigation. In this respect, human platelet lysate (hPL) has gained significant interest. A number of studies have shown that hMSCs expanded in flatbed cultures using hPL maintain their multipotency and therapeutic properties (6). And cells expanded in hPL have been used in clinical trials with no signs of significant adverse events. Thus, hPL is considered to be a safe alternative to FBS for expansion of hMSCs.
We investigated whether hPL could be used to expand hMSCs on microcarriers without affecting the cells’ identity and functionality. To that end, we cultured hMSCs on microcarriers and compared their expression of hallmark surface markers and various MSC-defining genes. Thus did we identify hPL as an alternative to FBS for microcarrier-based hMSC cultures.
Materials and Methods
hMSC Culture: Human mesenchymal stem cells were derived from bone marrow and cultured as described elsewhere (3). The culture medium consisted of Dulbecco’s modified Eagle’s medium (DMEM) from Life Technologies supplemented with either 10% MSC-qualified FBS (also from Life Technologies) or with 5% PLTMax supplement (Millcreek Life Sciences), 2 mM l-glutamine (EMD Millipore), 1× EmbryoMax penicillin– streptomycin solution (EMD Millipore), 8 ng/mL human recombinant basic fibroblast growth factor (bFGF) from EMD Millipore, and 2 U/mL heparin (Sigma-Aldrich). Collagen-coated microcarriers (Pall Corporation) were chosen for hMCs expansion in spinner flasks (Corning).
RNA Isolation, cDNA Generation, and QRT-PCR: We isolated RNA from hMSCs cultured in PLTMax or FBS-containing media using RNeasy mini kits from Qiagen, according to the protocol instructions or methods described elsewhere (3). Similarly, details of cDNA generation and quantitative reverse transcription polymerase chain reaction (QRT-PCR) also can be found elsewhere (3). Briefly, we isolated RNA from hMSCs cultured in PLTMax or FBS-containing media using RNeasy mini-kits from Qiagen, according to the protocol instructions. We used 1 ÎĽg total RNA for cDNA synthesis using qScript cDNA SuperMix solution (Quanta Biosciences). Real-time-polymerase chain reaction (RT-PCR) quantified relative gene expression using customer PCR array plates (SA Biosciences) and the CFX96 Touch real-time PCR system (Bio-Rad Laboratories).
Differentiation of hMSCs: We trypsinized the hMSCs grown in either PLTMax or FBS-containing media off their microcarriers and plated them onto 24-well tissue culture plates at a seeding density of
2.1 Ă— 104 cells/cm2 for inducing adipogenesis and a density of 4.2 Ă— 103 cells/cm2 for inducing osteogenesis, which is described elsewhere (3).
Fluorescence-Activated Cell Sorting: We resuspended the cell pellets in DMEM (Life Technologies) with 2% BSA (Sigma) to bring the cell concentration to 5 Ă— 105 cells/mL. Our detailed protocol is described elsewhere (3). Briefly, cells were read on a Guava easyCyte 8HT flow cytometry system (EMD Millipore) and raw data imported into the FlowJo program (Treestar) for analysis.
Results and Discussion
We recently identified collagen-coated microcarriers as well suited to expand hMSCs in stirred suspension cultures using standard medium containing FBS (3). To determine whether the same conditions would allow for expansion of hMSCs using hPL, we seeded cells directly onto collagen-coated microcarriers and cultured these in spinner flasks for several days.
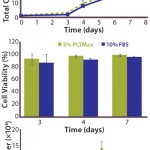
FIGURE 1: hMSCs cultured in 5% human
platelet lysate (hPL) showed similar expansion
in either a single culture with basic fibroblast
growth factor (bFGF) (top) or multiple passages
without bFGF (bottom) — and similar viability
rates (middle) — as cells grown in standard 10%
FBS-containing media conditions.
Expansion: As Figure 1(top) shows, cells grown for seven days in hPL showed similar expansion as those grown under standard, FBS-containing media conditions. Initial growth was slow under both sets of conditions for the first three days, which is likely due to seeding the cells directly after thawing, with no recovery phase in flatbed cultures first. However, the expansion rates from days four to seven in both sets of conditions were comparable. Furthermore, as Figure 1(middle) shows, cell viability was unaffected by culturing cells with hPL.
Interestingly, cells expanded in hPL on microcarriers showed no enhanced expansion compared with FBS-containing microcarrier cultures. Previous studies using flatbed cultures had shown that cells expanded with hPL displayed increased expansion rates despite the lower overall concentration of serum: 5% hPL rather than 10% FBS (6). Because hPL contains high concentrations of various growth factors, such as FGF2 and EGF, it is likely that such factors contribute to the enhanced growth observed in some hPL-containing cultures. In that context, it is noteworthy that the FBS-containing growth medium used in our studies was further supplemented by recombinant human FGF2. It is thus possible that the addition of FGF2 to FBS-containing medium can at least in part compensate for the potential advantageous effects of hPL for hMSC growth.
To be suitable for large-scale expansion in microcarrier-based cultures, hMSCs must be able to expand. That is made possible by adding fresh microcarriers to a bioreactor. Therefore, we explored the question of whether hMSCs grown with hPL on micorcarriers could be expanded through serial passaging.
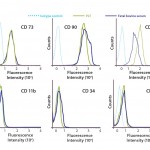
FIGURE 2: hMSCs cultured in 5% and 10% fetal bovine serum (FBS) media conditions retained their
identity as expression of cell-surface antigens.
We expanded hMSCs on collagen-coated microcarriers for seven days in the condition without bFGF as described (6). Then we transferred part of the culture (0.5 Ă— 106 cells) to a new spinner flask containing fresh medium and micorcarriers. As Figure 1(bottom) illustrates, we repeated the procedure to ensure continuous cell growth. Our serial expansion of hMSCs with hPL was comparable to the FBS-containing microcarrier cultures. So cells grown under both sets of conditions are able to sustain their growth rate and seed into fresh microcarriers even without the presence of bFGF.
Taken together, the above data show that hPL can be used for large-scale expansion of hMSCs on microcarriers.
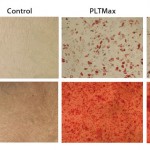
Figure 3A and 3B: hMSCs cultured in 5% and 10% fetal bovine serum (FBS) media conditions retained their identity through an ability for multipotent differentiation.
Multipotency: We next studied whether hMSCs expanded with hPL on microcarriers maintained their multipotent progenitor identity. Although no definitive marker for MSCs has been identified so far, some researchers have made efforts to define cell population through surface-marker expression as well as MSCs’ ability to differentiate into different cell populations. Dominici et al. published a summary of features that define MSCs (7). First, cells must grow adherent to a surface and express CD73, CD105, and CD90 on their cell surfaces. In addition, markers of hematopoietic cell lineages such as CD11b, CD34, and CD45 should not be expressed by MSC cultures. Finally, MSCs harbor the ability to differentiate along the adipose, osteogenic, and chondrogenic lineages.
Using those standard characteristics, we showed that hMSCs expanded for 21 days with hPL maintain their identity and potency (Figures 2 and 3). Microcarrier-expanded hMSCs display positive expression of CD73, CD105 and CD90, whereas no surface expression of CD11b, CD34 and CD45 could be detected (Figure 2). Furthermore, hMSCs expanded with hPL on micorcarriers maintained their ability to differentiate along the adipose and osteogenic lineages, showing that the cells maintained their multipotent identity after culture in hPL (Figure 3).
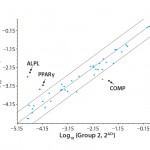
FIGURE 4: Polymerase chain reaction (PCR)–array assay showed that three out of 46 distinct genes related to hMSC identity and function appeared to be differently expressed in hPL-cultured and FBS-cultured cells.
To further evaluate the MSC identity of hPL-cultured cells, we performed a series of QRT-PCR assays for genes known to be related to MSC identity and function. Analyzing the expression of 46 distinct genes, we identified three in particular that appear to be differently expressed in hPL-cultured and FBS-cultured cells (Figure 4). Whereas the osteocyte and adipocyte lineage marker genes for alkaline phosphatase (ALPL) and peroxisome proliferator-activated receptor gamma (PPARÎł) display an increase in mRNA expression levels, the expression of the chondrocyte lineage marker, cartilage oligomeric matrix protein (COMP), is reduced in hPL-cultured cells. However, because differentiation along the osteogenic lineage is not affected by those changes (Figure 3) and the expression of additional marker genes is not affected (Figures 2 and 4), it is unlikely that culture of hMSCs with hPL leads to a loss of multipotency and onset of adipocytic differentiation.
An Attractive Substitute
Our data demonstrate the utility of hPL for large-scale expansion of hMSCs in microcarrier-based cultures. We found that hMSCs expanded in hPL maintain their MSC identity and potency similar to previous observations using flatbed cultures. Thus, hPL offers a viable alternative to FBS as a serum supplement for large-scale microcarrier-based hMSC cultures. With recent cell therapy successes, process development for large-scale manufacturing of cells is of increasing interest. So the combination of hPL with microcarrier-based cultures will be an attractive opportunity.
Acknowledgments
Preparation of this publication required efforts of the following personnel at EMD Millipore Corporation: Daniel Kehoe and Michael Phillips.
References
1 Rowley J, et al. Meeting Lot-Size Challenges of Manufacturing Adherent Cells for Therapy. BioProcess Int. 10(3) 2012: 16–22.
2 Jing D, et al. Characterization of Human Mesenchymal Stem Cells: Expansion in a 3-L, Single-Use, Stirred-Tank Bioreactor. BioProcess Int. 11(3) 2013: 30–36.
3 Jing D, et al. Growth Kinetics of Human Mesenchymal Stem Cells in a 3-L Single-Use, Stirred-Tank Bioreactor. BioPharm Int. 26(4) 2013: 28–38.
4 Kehoe D, et al. Scale-Up of Human Mesenchymal Stem Cells on Microcarriers in Suspension in a Single-use Bioreactor. BioPharm Int. 25(3) 2012: 28–38.
5 Bieback K. Platelet Lysate as Replacement for Fetal Bovine Serum in Mesenchymal Stromal Cell Cultures. Transfus. Med. Hemother. 40(5) 2013: 326–335.
6 Crespo-Diaz R, et al. Platelet Lysate Consisting of a Natural Repair Proteome Supports Human Mesenchymal Stem Cell Proliferation and Chromosomal Stability. Cell Transplant. 20, 2011: 797–811.
7 Dominici M, et al. Minimal Criteria for Defining Multipotent Mesenchymal Stromal Cells: The International Society for Cellular Therapy Position Statement. Cytother. 8(4) 2006: 315–317. •
Sandhya Punreddy is a research scientist III in the stem cell bioprocess group at EMD Millipore, 80 Ashby Road, Bedford, MA 01730; 1-781-533-5671; sandhya.punreddy@emdmillipore.com. At the time this paper was written, Knut Niss was an R&D manager at EMD Millipore, Donghui Jing, PhD, was a research scientist III in the stem cell bioprocess group at EMD Millipore, and Neethu Sunil was a research scientist at Experis.
mesenchymal stem cells (MSCs) have been scrutinized and studied, garnering much attention due to their broad therapeutic efficacy. In Mesenchymal Stem Cells: Methods and Protocols, leaders in the field were assembled to contribute detailed methodologies for the isolation and characterization of human and rodent MSCs.
More information at http://www.creative-diagnostics.com.
MSCs have a vital role in tissue engineering and cell therapy (often referred to as regenerative medicine). They are able to modulate the immune system which can facilitate tissue repair through the secretion of cytokines, chemokine, growth factors and signalling molecules into the medium. MSCs are also able to differentiate into chondrocytes, osteoblasts and adipocytes, the immune properties gives them an important role in treating many immunological disorders. MSCs can be identified in many different tissue beside the marrow cavity such as adipose tissue, muscle, blood, dental pulp, dermis, perivascular areas, umbilical cord blood, tissue’s surrounding the umbilical cord vessels, placenta and amniotic fluid.