Cell line development engineers in the biopharmaceutical industry juggle several, sometimes contradictory priorities. They must present their bioprocessing colleagues with a master cell line that can express a reproducibly high-quality protein product at titers and growth concentrations that will be high enough for manufacturing efficiency — and without those parameters degrading over time. Performing the first step in every bioprocess, these scientists must consider their own budgetary concerns and efficiencies while facing regulatory scrutiny under the 21st-century risk-management paradigm. In turn, they make demands on technology suppliers, asking, “Can we engineer our cell lines to obtain good productivity and genetic stability without screening so many clones? How do we best manage those screenings? What properties can we screen for? And how important is clonality, anyway?†The answers must come from biology enabled by improved instrumentation.
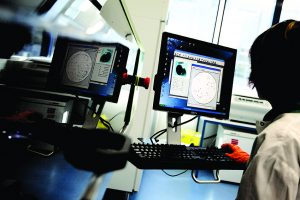
This laboratory at cell-therapy company Cellectis is one of >100 worldwide using the established ClonePix colony picking technology from Molecular Devices LLC. (WWW.CELLECTIS.COM, WWW.MOLECULARDEVICES.COM)
At KNect365’s “Cell line Development and Engineering†meeting (CLDE) in San Francisco this past June, Susan Sharfstein of the State University of New York’s (SUNY’s) Polytechnic Institute addressed some of those questions. She illustrated the phenomenon of cellular adaptation, which to me seemed to contract the value of “genetic stability†as a goal.
“All the cell lines we had (which were given to us by a biotech company and represent a relatively late stage in the cell line development process) are pretty stable,†she explained to me later, “at least when it comes to expression.†But these cell lines can adapt to increased productivity based on a number of factors: transcriptional/translational efficiency, messenger RNA stability; and protein stability, folding, and secretion.
Sharfstein’s laboratory is using DNAse footprinting, proteomics, genomics, and transcriptomics to classify genes according to their functions and shared pathways as a means of elucidating basic information about productivity and stability. Increased productivity generally is accompanied by widespread changes in cellular physiology. Different clones show varied activation of transcription factors. So a measure of genetic “instability†is to be expected as an engineered cell line is optimized. And true, rigid stability may not be what developers really want — at least not during development.
Most of the data Sharfstein presented came from a proteomics collaboration between her laboratory and one at Dublin City University. She looks forward to further studies and quantitative work based on clustered regularly interspaced short palindromic repeats (CRISPR) and Cas9 genome-editing technology. The resulting science will help drive technologies for bioprocess engineers to develop the most efficient and robust cell lines that they can. This echoes the theme of the conference overall: Advancing technologies are enabling both researchers and bioengineers to gather the necessary data they need to work better, faster, and smarter than ever before.
The Conundrum of ClonalityÂ
A 1997 guidance document from the International Council on Harmonisation of Technical Requirements for the Registration of Pharmaceuticals for Human Use (ICH) urges biomanufacturers to begin each process with a clonal cell substrate — derived from a single cell progenitor (1). As technologies have advanced, regulators have increased their scrutiny of cell line development with clonality in mind. What once was a hands-on practice of repeated dilution to isolate cells became the realm of flow cytometry, fluorescent imaging, and increasingly smaller-scale laboratory culture instruments. Scientists now have the ability to document precisely the single-cell origin of a cell line — although many now question the need to do so.
The intention behind this focus on clonality is to characterize bioprocess reproducibility. Regulators want to see production of each successive biopharmaceutical batch/lot coming from genetically identical cells to those of previous lots. Such an idea naturally falls in line with the 21st-century focus on risk management and quality by design (QbD). But advancing science is showing that true genetic stability may well be impossible.
At the CLDE conference, Martina Baumann of the Austrian Center of Industrial Biotechnology showed how chromosomal rearrangement is common with rapidly growing cell lines such as those used in bioprocessing. Her team found that different host-cell lines are distinguishable by marker chromosomes and that both the numbers and karyotypes of those chromosomes vary within a cell population. In fact, different CHO lines exhibit a range in their modal number of chromosomes present — from 18 to 22, with the Chinese hamster itself having 22. By comparison, all the billions of humans on Earth have the same number of chromosomes.
CHO cells enjoy a prominent position in the biopharmaceutical world because they are easy to manipulate genetically, adaptable to serum-/protein-free media and large-scale suspension culture, robust to changes in culture conditions, relatively insusceptible to human pathogens, and capable of performing human-like glycosylation of protein molecules. However, with those advantages come challenges related to genomic/phenotypic instability, potential decreases in productivity and product quality, problems with reproducibility, and financial issues related to (among other things) the labor associated with long development and culture time lines related to those of microbial hosts.
“Genomic variance occurs with each division,†Baumann explained.
As Pfizer’s Jack Scarcelli reported later, data from three major biopharmaceutical companies — Amgen, Pfizer, and Genentech (Roche) — showed significant heterogeneity from clonal cell lines. Such lines can and do perform consistently in upstream biomanufacturing and generate materials of consistent product quality. In fact, single-cell derivation alone is not a good predictor of cell line consistency.
Scarcelli pointed out that single-cell cloning remains important, especially when random-integration genetic engineering platforms are used. But it doesn’t guarantee genetic/phenotypic homogeneity. To ensure that cell-bank heterogeneity won’t compromise process consistency (robustness) and that cells are suitable for commercial manufacturing, he said, “it is good practice to assess cell banks both genetically and phenotypically in a representative process across generational age.â€
His Pfizer colleague Karin Anderson showed that heterogeneity isn’t always bad. The adaptability that made CHO cells the workhorses of bioprocessing brings a genomic plasticity. “Clonality is important, but what is most important is process robustness,†she said. “And heterogeneity is what gives us that.â€
The more cell divisions happen as a culture ages, the more mutations will occur and the more heterogeneous that culture becomes — particularly when conditions change. From a single-cell clone to inoculation into a 12,000-L bioreactor, Anderson illustrated, a cell line has doubled 100 times — with 6.34 × 1029 individual cell divisions! Each division has a chance to introduce mutations that will be carried on.
She showed how sequence variants can be used as genetic markers to study population dynamics in clonally derived cell lines. Populations that seem homogenous do demonstrate genetic heterogeneity. But those with undetectable differences in growth rate can outgrow others — ultimately leading to a more “homogenous†cell line through traditional evolution. Highly homogenous cell lines are more susceptible to changing process parameters. And although heterogeneous lines can contain subpopulations with undesirable attributes, that mixture of geno-/phenotypes also protects the overall culture. Identifying genetic markers that correlate with unwanted characteristics allows for early screening and exclusion of those cells.
Cloning enables selection of cells with desirable attributes; cell line engineering can eliminate the undesirable. But in general, Anderson said, “the right kind of heterogeneity generated by cell division contributes to a robust and consistent process.â€
A single cell clone gives a definable starting point to a given bioprocess — a means to begin the process of mutation back at “square one†from a previously cultured immortalized cell line. Coming from that single clone, the pools of cells that follow for screening will proceed with their natural mutations and gene shuffling over time through optimization of media and other culture conditions. But those cells will share a common origin — what regulators want to see.
Rise of the Machines
Platforms: A number of CLDE presentations featured instruments designed to help companies demonstrate that. For example, Solentim’s Ian Taylor described his company’s Verified In Situ Plate Seeding (VIPS) system that combines single-cell printing and whole-well imaging — an alternative to fluorescence-activated cell sorting (FACS).
Kim Le of Amgen described his company’s goal of “digitalizing†cell line development through nanoliter miniaturization, automation, and data management. Amgen is working with the Beacon system from Berkeley Lights, which corrals cells on laboratory chips. Like many other speakers, Le pointed out that “a clone is only a clone until it divides.†His group considers single-cell methods to be key to demonstrating clonality.
Of course, these methods must be validated to work well and reproducibly. Anke Mayer-Bartschmid of Bayer focused on how to do so with single-cell imaging systems. She presented ongoing work from a BioPhorum Development Group (BPDG) committee comparing the results of limiting dilution, cell sorting, and single-cell plating methods while assessing the confidence level of imaging systems. “Combining one round of limiting dilution or a qualified FACS or other single-cell deposition method,†she said, “with high-throughput single-cell imaging can demonstrate monoclonality with more reliability than the current standard two rounds of limiting dilution.â€
In a tandem talk, John Yan of Prozyme and Divya Goel of Aetos Biologics presented a case study in applying rapid sample-preparation workflows for elucidating the structure of N-linked glycans, which is important to the pharmacology of biotherapeutics (2–5). Characterization of those glycans thus is vital to development of all glycosylated biotherapeutics.
Prozyme’s GlykoPrep platform combines reagents, analytical methods, and automation. Goel emphasized the need for rapid results to make decisions about cells that are aging and growing while scientists wait for sample preparation and analysis. This is especially important for biosimilar developers trying to reproduce innovators’ processes as well as they can. Using Prozyme methods, Aetos screened and identified top biosimilar clone candidates and optimal processes in under four months (from transfection), then demonstrated process scalability and reproducibility from 3-L to 50-L bioreactors. Goel said her company reduced labor; increased sample throughput; reduced the burden, risk, and timeline of process optimization; and ultimately sped up development.
Automation, Streamlining: Automation can speed up operations in many aspects of biopharmaceutical development including cell line development. We see microliter-, nanoliter-, and now even picoliterscale lab-on-a-chip work providing cell line engineers with advanced options for demonstrating clonality and characterizing the expression capabilities of single cells.
David Shaw of Genentech (a member of the Roche Group) described his company’s use and characterization of single-cell printing. “Automated single-cell cloning (SCC) and hit-picking workflows have been in use for several years,†he explained. “They use plate imaging to document that our cell lines are clonally derived. We added single-cell deposition to our plate-imaging workflow to gain significant efficiencies over limiting-dilution SCC. We use existing hit-picking automation to pick and scale up single-cell clones, then developed custom seed-train automation to set up and sample fed-batch cultures in an unattended operation.†This workflow helps his team meet its projected needs of developing 50–200 cell lines each year with a very limited staff.
David Auslander of Novartis also presented a sped-up workflow. He described the requirements of a biomanufacturing cell line: high productivity of adequate proteins, long-term relative stability and monoclonality, good platform fit, and a fully documented history. Then he contrasted the established means of achieving those in 14–18 weeks — from initial transfection to generate a pool of cells exhibiting the gene of interest through cloning, expansion, and selection, to productivity improvements in microscale culture — with his company’s fast-track process. After transfection, aggressive pool generation produces high-quality clones that go through a FACS vector-based process of expansion and preselection for microscale culture productivity work — all in eight weeks.
Biogen’s James Lambropoulos also described a streamlined workflow — in this case cutting down the number of assays his team traditionally performed. “Rational curation of product-quality data led to a 30% reduction in the number required,†he summated. “A deep-well screen can predict some product-quality attributes but not ultimate titer rankings. Dramatic timeline savings require dramatic workflow changes: new scale-down systems and targeted integration.â€
Laboratories need to produce and characterize many cell lines, not only for biopharmaceutical production, but also in support of analytical studies. Elizabeth Scheideman of the National Institute of Allergy and Infectious Diseases (part of the US National Institutes of Health) reported on vaccine-related transient transfection and cell line development using high-throughput and FACS technologies. She described timeline improvements for transient transfection (down to about two weeks), stable pool generation (in about six weeks), and clonal cell line production of 3 g/L protein (in about four months).
Amgen’s Richard Altman focused exclusively on transient transfection, often used to make small amounts of reagent proteins quickly for drug-discovery assays. He reviewed his team’s evolving protein production endeavors by focusing on strategic assembly of genetic “tools†that help cells express diverse and challenging candidate proteins; and harmonized production processes that reduce turnaround times while increasing throughput. Altman emphasized that there is no “one-size-fits-all†solution, especially with growing demand for an expanding range of recombinant proteins. His group has shortened expression time lines by about half.
Making Molecular Improvements
University of Manchester professor Alan Dickson challenged the audience with questions that can be answered only now that cell line development and characterization technologies offer options such as the CRISPR/Cas9 system. “How do we select the appropriate genes to edit? Background literature suggests some that are obvious, but the multiplicity of interactions in the environment of the cell makes this unpredictable.†Using model CHO cell systems, Dickson highlighted approaches and technologies that screen for genes representing the “drivers†controlling CHO-cell suitability for bioproduction.
He offered epoetin as an example of a difficult-toexpress protein in a case study using the commercial Tet-On inducing system (Clontech Laboratories), the T-REx CHO cell line (Thermo Fisher Scientific), and an nCounter instrument (NanoString Technologies). Results suggested “families†of genes based on expression pathways they seem to share in responding to the same stressors. Controlling for functional outcomes is not as simple as cause-and-effect because gene-modifiers affect them as do other, secondary outcomes along the way. To address related technical challenges, Dickson recommends multiplex-targeting genetic engineering and systems-based analysis of correlative/causal relationships that determine cell stability (6).
The questions that cell line developers now can ask include, “What does recombinant protein expression do to cells? What might enhance production without compromising stability and robustness? Can we guess a target gene from what we know about its ‘family,’ stressors, and desired outcomes?†To date, Dickson said, developers have focused on targets that follow from what is known. Real “chassis engineering†of cell lines will require more knowledge.
Several presenters reported using advanced technologies to identify relevant sequences and engineer genomic improvements to cell lines in development. For example, Genentech’s Shahram Misaghi highlighted control of ubiquitin, a protein related to proteolytic degradation in mammalian cells, as a means of improving production titers. ATUM’s Ferenc Boldog focused on the use of transposase enzymes and their cognate transposons in rapid development of highly productive, stable CHO and other mammalian cell lines. Kevin Kellner of Dublin City University presented improvements to biopharmaceutical production using microRNAs as targeted genome engineering tools. And Genentech’s Benjamin Haley reported on a CRISPR/Cas9 platform development for drug-target discovery and validation.
Nathan Lewis of the University of California at San Diego highlighted that system’s speed. “Genome-sequencing efforts have provided a parts list for CHO cells,†he said. “CRISPR has enabled rapid engineering.â€
But Horizon Discovery’s Aleksandra Jaskot pointed out that using CRISPR with CHO cells was difficult initially. It has taken a few years to design the necessary libraries. Meanwhile, “aside from process improvements focused on media and feed strategies,†she said, “the CHO host remains largely unchanged from what was used 30 years ago.†Her company is improving it while taking manufacturability and the intellectual property (IP) landscape into consideration when designing vectors and making related cell line development decisions. The goal is to provide clients with the freedom to operate that won’t be inhibited by litigation down the line.
Biogen’s Eric Lee described his company’s “toolkit†for balancing productivity and product quality (7). The team uses a double-knockout vector and amplification method based on zinc-finger nuclease transfection for stringent selection in screening clones and pools of cells. “Behind every great cell line,†Lee pointed out, “is a great host cell.†Stringent screening can speed up the path from one to the other.
Genetic Changes: Merck’s Benson Li pointed out that genetic stability of highly adaptable CHO cells is a perennial concern. He suggested several means to address that: discovering and predicting instability issues early on, using targeted-integration genetic engineering methods, and improving vector designs. “It is critical to demonstrate cell line phenotypic stability and product-quality consistency before master cell banks are generated — covering population doubling levels expected for commercial manufacturing. Genetic characterization of top clones should be performed as soon as feasible. Investigate promptly to understand the root cause of instability.â€
AbLab’s Anton Bauer described how his company developed stable, high-yield production cell lines “at unprecedented speed†using chromosomal loci in bacterial artificial chromosomes (BACs) with random integration into mammalian host-cell chromosomes. “Having the gene of interest embedded in the defined locus protects from negative host chromatin effects,†he said, “and integrating multiple copies of this large expression unit correlates with high specific productivity.†The group has generated production clones for antibodies and even difficult-to-express proteins within three weeks from transfection.
Amgen’s Cai Guo highlighted challenges in producing bispecific antibodies — and how vector design affects protein structure and quality. Using charge-pair technology helps cells assemble bispecifics correctly, but expression imbalance and/or structural mispairing can yield undesirable product variations. Guo’s team combines genomic engineering, crossfunctional molecular screening, cell line selection strategies, and prediction tools to reduce that product heterogeneity.
Using Feeds to Trigger Productivity: Each cell line responds best to its own particular means of transcription and culture conditions. Although new technologies are helping cell line developers engineer and screen pools and clones, the always-complex IP landscape of biotechnology can present obstacles that have little to do with science. Even so, the number of available options has expanded to allow more specificity than ever in choosing what works best for a given project. And even as it becomes possible to tinker with cells on a genomic level, that doesn’t mean laboratories will abandon more traditional optimization methods — especially when new science has improved those as well.
In his CLDE talk, Yves Durocher of Canada’s National Research Council described an expression platform for rapid production of recombinant proteins. “We use a cumate-inducible CHO expression system that generates stable and scalable pools expressing high levels of recombinant proteins in two to three weeks. The pools are also used to derive stable and high-expressing CHO clones for manufacturing therapeutic candidates.â€
Noting that “high expressers often show the slowest growth,†he explained that cell line engineers must determine how to get the most material soonest by balancing those parameters. Inducing systems start production only when it’s needed — turning it off for higher growth and on for production with culture ingredients such as tetracycline antibiotics and often based on knock-in or knock-out genes (8–11). An inducer, Durocher pointed out, should be nontoxic and cost-effective as well as easily removed by downstream processing — and cumate satisfies those requirements. In NRC experiments, this system outperformed others from transfection to >10-L harvest in four to five weeks.
Boaz Tirosh of Hebrew University optimized culture media by adding a cadmium chloride at low concentrations to improve expression (12). Cadmium is toxic to cells at high concentrations (e.g., 5 µM), but when present at ~0.5 µM concentration, it can improve protein expression and production. In fact, Tirosh said, even with cadmium at toxic levels the cells that survive express proteins at much higher levels than before it was introduced. His team determined how to balance cell die-off against that higher production.
Bayer’s Mark Trautwein described a new stringent-selection method using an amino acid as a metabolic marker. “High-stringency selection is well known, but many existing solutions exhibit different drawbacks.†His group identified an endogenous marker in CHO cells: their inability to synthesize proline. Without proline added to culture media, most CHO cells die within 10 days. So adding a synthase enzyme to cultures helps select for stably transfected cells in proline-free medium — a method that could be applied to all CHO cell lines without genetic modification. Trautwein emphasized that product quality cannot be compromised, and his team has yet to see negative effects to it using this system.
Engineering Solutions
In one keynote presentation, the University of Sheffield’s David James described “new parts and systems for CHO cell synthetic biology.†He said that the common approach to cell line development — screening cells “in hopes of finding a good clone†— doesn’t pass muster according to the classic design–build–test–learn cycle of engineering. Many cell line developers are missing the latter two aspects completely, and James showed how modernizing technologies and strategies could help.
“Synthetic biology makes it possible to engineer host cells,†James explained, “through combinatorial ‘tuning’ of discrete cellular synthetic processes and directed engineering of the synthetic capacity and process performance of the host cell itself. Using this approach permits ‘one-size-fits-all’ genetic vectorology, replacing mechanistically blind screening of transfected cells with tailored design and construction of specifically fit-for-purpose cell factories. This system relies on a toolbox of synthetic ‘parts’ with user-defined functionality and platform technologies that enable product manufacturability.†Combining genomic, transcriptomic, and proteomic data with computer modeling (13) could enable bioengineers to create new genetic parts and cell engineering strategies that control core processes such as genetic transcription/translation and polypeptide folding/assembly in what James calls “a high-capacity host-cell chassis.â€
That describes perhaps the ultimate destination of the path on which cell line developers find themselves now. When knowledge drives advancement, biology can drive biotechnology, and mere development becomes true engineering. Thus, in silico design of genetic constructs can lead to building cells in vitro with high-throughput gene synthesis, live testing with high-throughput expression and analysis, and computational learning that will in turn drive further design improvements in the engineering cycle. The typical cell line development workflow begins with genetic vectors and transfection of a chosen host cell culture, followed by selection and amplification of correctly transfected cell pools. Those get screened according to a range of parameters such as productivity and robustness before subclone isolation, screening, and clonality assurance.
The final chosen clone then serves as progenitor to a new and unique cell line for producing a given protein product. Cell-banking follows for ultimate seed-train scale-up into production bioreactors. From transfection to induction to screening, the options available to scientists responsible for delivering that final master cell bank to upstream biomanufacturing groups have increased dramatically in the 21st century so far. And with academics like those presenting at CLDE continuing to push the science forward, there appears to be no end in sight to the levels of specificity possible as innovators and biosimilar makers work to develop that perfect cell line for every biopharmaceutical product.
A Sponsored eBook from BPI |
At KNect365’s European Cell Line Development and Engineering conference — April 2018 in Amsterdam, The Netherlands — cytena CEO Jonas Schöndube gave a presentation highlighting some of his company’s recent developments in single-cell dispensing for documenting clonal cell lines. Based on that talk, this eBook discusses how increasing throughput with the use of higher density 384-well plates and efficient single-cell isolation techniques saves time and cost as the industry moves from reusable components to disposables. |
Download the eBook at www.bioprocessintl.com/sponsored-content/assurance-of-clonality-next-generation-single-cell-dispensing-in-cell-line-development-and-single-cell-genomics. |
References
1 ICH Q5D. Derivation and Characterization of Cell Substrates Used for Production of Biotechnological/Biological Products. US Fed. Reg. 63(182) 1998: 50244-50249; www.ich.org/fileadmin/Public_Web_Site/ICH_Products/Guidelines/Quality/Q5D/Step4/Q5D_Guideline.pdf.
2 Jones A. N-Glycan Analysis of Biotherapeutic Proteins. BioPharm Int. 30(6) 2017: 20–25.
3 Higel F, et al. N-Glycosylation Heterogeneity and the Influence on Structure, Function and Pharmacokinetics of Monoclonal Antibodies and Fc Fusion Proteins. Eur. J. Pharm. Biopharm. 100, 2016: 94–100; doi:10.1016/j.ejpb.2016.01.005.
4 Liu L. Antibody Glycosylation and Its Impact on the Pharmacokinetics and Pharmacodynamics of Monoclonal Antibodies and Fc-Fusion Proteins. J. Pharm. Sci. 104(6) 2015: 1866–1884; doi:10.1002/jps.24444.
5 Abes R, Teillaud J. Impact of Glycosylation on Effector Functions of Therapeutic IgG. Pharmaceut. 3(6) 2010: 146–157; doi:10.3390/ph3061887.
6 Maldonado-Agurto R, Dickson AJ. Multiplexed Digital mRNA Expression Analysis Profiles: System-Wide Changes in mRNA Abundance and Responsiveness of UPRSpecific Gene Expression Changes During Batch Culture of Recombinant Chinese Hamster Ovary Cells. Biotechnol. J. 13(3) 2018; doi:10.1002/biot.201700429.
7 Wright et al. Leveraging a CHO Cell Line Toolkit to Accelerate Biotherapeutics into the Clinic. Biotech. Progr. 33(6) 2017: 1468–1475; doi:10.1002/btpr.2548.
8 Jones J, et al. Optimization of Tetracycline-Responsive Recombinant Protein Production and Effect on Cell Growth and ER Stress in Mammalian Cells. Biotechnol. Bioeng. 91(6) 2005:722–732; doi:10.1002/bit.20566.
9 Misaghi S, Chang J, Snedecor B. It’s Time to Regulate: Coping with Product-Induced Nongenetic Clonal Instability in CHO Cell Lines Via Regulated Protein Expression. Biotechnol. Progr. 30(6) 2014; doi:10.1002/btpr.1970.
10 Lam C, et al. Taming Hyperactive hDNase I: Stable Inducible Expression of a Hyperactive Salt- and Actin-Resistant Variant of Human Deoxyribonuclease I in CHO Cells. Biotechnol. Progr. 33(2) 2017: 523–533; doi:10.1002/btpr.2439.
11 Poulain A, et al. Rapid Protein Production from Stable CHO Cell Pools Using Plasmid Vector and the Cumate Gene-Switch. J. Biotechnol. 255, 2017: 16–27; doi:10.1016/j. jbiotec.2017.06.009.
12 Mohameed M, Tirosh B. Engineering CHO Cells with an Oncogenic KIT Improves Cell Growth, Resiliance to Stress, and Productivity. Biotechnol. Bioeng. 114(11) 2017: 2560–2570; doi:10.1002/bit.26356.
13 Macdonald JT, et al. Computational Design Approaches and Tools for Synthetic Biology. Integrat. Biol. 3(2) 2011: 97–108; doi:10.1039/c0ib00077a.
Cheryl Scott is cofounder and senior technical editor of BioProcess International, PO Box 70, Dexter, OR 97431; 1-646-957-8879; cscott@bioprocessintl.com.