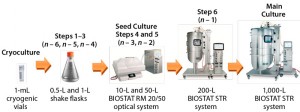
Figure 1: Seed train of the 1,000-L fed-batch cell culture run starts with one cryogenic vial before six consecutive cell-expansion steps using single-use shaker flasks and bioreactors. A 17-day fedbatch production process followed in a BIOSTAT STR 1000 system. The entire duration from cryogenic vial to 1,000-L harvest on day 17 took 35 days.
In the past decade, single-use bioreactors have gained wide acceptance for biomanufacturing. The biopharmaceutical industry is increasingly interested in performing modern production processes in single-use facilities. That trend is driven by the time and cost benefits of single-use technologies, as well as the enhanced manufacturing flexibility they offer (1).
With single-use bioreactors increasingly used in late-phase clinical trials and commercial production, their quality, reliability, and assurance of supply becomes more critical. Many industry experts consider process control of film and bag manufacturing and traceability of raw materials to be key to ensuring batch-to-batch consistency with single-use bioprocessing bags. Especially crucial for such bags is excellent and consistent cell-growth performance for a broad range of different commercially relevant cell lines.
Most commercially available film materials used for single-use bioreactors today were not specifically developed for cell culture applications. Therefore, Sartorius Stedim Biotech partnered with Südpack (Europe’s leading film-extrusion company) and closely collaborated with resin and additive suppliers to develop a new polyethylene film (“S80”) specifically optimized for such applications (2, 3). This collaborative concept enabled us to develop a completely new range of bioprocessing bags that meet industry needs for future biomanufacturing: consistent cell-growth and extractable profiles, superior robustness and ease of use, unprecedented assurance of supply, and suitability for all applications from upstream production to downstream processing and final filling (3–5).
The S80 polyethylene film used in Flexsafe bags has an optimized additive package controlled by specifications rather than trade names of resins and additives. Film extrusion, bag assembly, and γ-irradiation are all controlled within established process parameter ranges. This approach based on specifications and controls prevents detrimental effects on cell growth that can be caused by extractables released by film materials during bag manufacture and storage (3). Measured by a Chinese hamster ovary (CHO) cell–based cell-growth assay developed by Sartorius Stedim Biotech, the new film has demonstrated superior biological performance in accelerated aging studies and media-storage trials (3).
Similarly excellent results were recently published by the “Single-Use Technology in Biopharmaceutical Manufacturing” temporary working group (4). In their study, nine different cell lines and related media were used bioprocessing bags from different vendors with regard to their ability to support cell growth.
In addition to biocompatibility, the robustness of Flexsafe bags has been confirmed through a number of different test methods, including an in-house water-burst test and worst-case application trials (2, 5). Finally, functionality and consistent, reproducible performance of these Flexsafe bags has been qualified following industry accepted methods (2).
In the study reported below, we discuss the performance of Flexsafe STR bags in a high–cell-density fed-batch culture of recombinant CHO cells producing a monoclonal antibody (MAb). Fed-batch cultures ran in 50-L and 1,000-L BIOSTAT STR single-use, stirred-tank bioreactors for 17 days, with the results compared with those obtained using a 5-L BIOSTAT B stirred-tank glass bioreactor. This fed-batch process is considered to be a suitable model system for process verification of Flexsafe STR bags regarding cell growth, productivity, and robustness in typical customer cell culture applications because such processes using CHO cells are widely used in the biopharmaceutical industry (7). The recombinant CHO cell line we used is the same one involved in the cell-growth assay our company established to support development of the new polyethylene film (8).
Process Verification
We applied a recombinant CHO DG44 DHFR– cell line from Cellca GmbH to produce an IgG1-type MAb in a high–cell-density fed-batch process. Final MAb titers >7 g/L are typically reached with this cell line. We fed cultures using the chemically defined ActiCHO cell culture media system developed by Cellca GmbH: ActiCHO SM for cell expansion and ActiCHO P for production, with ActiCHO Feed-A, ActiCHO Feed-B, and a 400-g/L d-glucose solution from Sigma Aldrich.
The seed train for the 1,000-L fed-batch production process consists of six consecutive cell-expansion steps (Figure 1) starting with cryogenic vials. The inoculum cell density of all seed train steps was 0.2 × 106 cells/mL. For the first three expansion steps, we used disposable shaker flasks (n – 6, n – 5, and n – 4) incubated in a CERTOMAT CTplus CO2 incubation shaker. We further expanded the cells in 5-L (n – 3) and 25-L (n – 2) working volumes using a BIOSTAT RM rocking-motion bioreactor. The last seed expansion step — at 200 L in a BIOSTAT STR 200 (n – 1) system — generated the required inoculum volume of ~100 L for the large-scale run. All single-use bioreactors used in our study were equipped with Flexsafe bags.
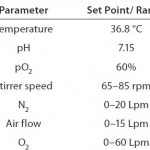
Table 1: Cultivation conditions of the 1,000-L
production process comprising set points for
pH, pO2, and temperature and including
ranges of gas flow rates and stirrer speeds as
part of the pO2 control cascade
The 1,000-L production process started with a three-day batch phase followed by a 14-day fed-batch phase. We inoculated a BIOSTAT STR 1000 production bioreactor at an initial cell density of 0.3 × 106 cells/mL. Table 1 lists the cultivation conditions set for the production bioreactor. We used two three-blade segment impellers and a ring sparger based on a previously performed characterization study on the bioreactor system, which suggests sufficient oxygen transfer using that set-up (9). As Table 1 shows, the system controlled pO2 in a cascade-control approach involving stirrer speed and sparging with nitrogen (N2), air, and oxygen (O2). Carbon dioxide (CO2) sparging controlled pH levels.
Superior Biological Performance at All Scales
The main aim of our study was to confirm the suitability and performance of Flexsafe bags for their final process application in both rocking-motion and stirred-tank formats up to the 1,000-L scale. Previous studies had already confirmed that the S80 polyethylene film material ensures superior and reproducible cell growth based on medium extraction trials using test bags (3). So our focus was to demonstrate not only that the film delivers excellent growth results, but also that the entire bag assembly performs well in a true and representative process application. During seed expansion (n – 3 to n – 1) using the BIOSTAT RM 10 (n – 3) and 50 (n – 2) as well as in the n – 1 BIOSTAT STR 200 seed bioreactor, we observed typical growth behavior that was comparable to what we have seen in cell expansions using shaker flasks. At the end of the three-day batch growth phase, viable cell densities (VCDs) of ~2 × 106 cells/mL with viabilities >99% were reached (data not shown).
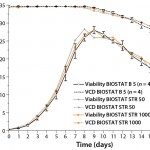
Figure 2: Viable cell density (VCD) and viability of high–cell-density fedbatch
processes in a BIOSTAT B 5-L glass vessel and BIOSTAT STR 50 and BIOSTAT STR 1000 Flexsafe bags; 5-L data include a standard deviation of cell density and viability for a total of four runs.
Figure 2 compares cell growth and viability data of the high–cell-density fed-batch run at 1,000-L scale with data from 50-L scale using the single-use BIOSTAT STR bioreactor set-up. Our large-scale, single-use, bag-based bioreactors achieved excellent comparability in both runs. Those results are further supported by excellent comparability seen with small-scale reference data generated during four 5-L cultures in BIOSTAT B glass vessels. At 5-L, 50-L, and 1,000-L scales, all cultures achieved peak cell densities of ~28 × 106 cells/ mL at >97% viability (measured by a Cedex HiRes cell-counting system from Roche Diagnostics) on day 9 post inoculum. Growth and viability profiles follow each other very closely at all scales. Notably, cell growth data from the BIOSTAT STR 50 and 1000 were within the standard deviation of the data from 5-L reference runs using conventional glass stirred-tank vessels. Hence, we observed no cell-growth–inhibiting effects with Flexsafe bags in the large-scale, stirred-tank format, which demonstrated excellent comparability and scalability of the bioreactor systems.
Accelerated aging studies of sample bags showed no impact on biological performance of the S80 film formulation during an investigated 36-month shelf-life period (3). Our study involved Flexsafe RM and STR bags that had been stored between one and 12 months. The consistent and superior cell growth obtained with the different scales and bioreactors further confirms those accelerated aging data using sample bags.
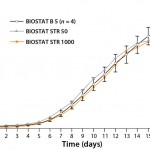
Figure 3: Monoclonal antibody (MAb) titer from high–cell-density fedbatch
processes in a BIOSTAT B 5-L glass vessel and BIOSTAT STR 50 and BIOSTAT STR 1000 Flexsafe bags; 5-L data include a standard deviation of product titer for a total of four runs.
In addition to cell growth and viability, product formation (in this case an IgG1 MAb) is especially critical to establishing comparability of different cell culture systems. Figure 3 shows that, at all investigated scales, IgG1 titers were highly comparable and within the standard deviation of the 5-L glass vessel results. A final MAb titer of 7.8 g/L was achieved with the high–cell-density fed-batch run at 1,000 L, which is comparable to 8.2 g/L achieved at the 50-L scale. Specific production rates ranged from 11 ng/cell on day 1 to 45 ng/cell on day 13. We used reduced sodium-dodecyl sulfate polyacrylamide gel electrophoresis (SDS-PAGE) to analyze product purity and potential degradation at different culture days of the 1,000-L high–cell-density fed-batch run and compared the results with an IgG1 control (Figure 4). Product-specific bands found at ~25 kDa and 50 kDa showed consistency with the control. Moreover, we discovered no significant impurities. Based on those data, we excluded the possibility of detrimental effects on productivity with Flexsafe bags. Negative impacts on product purity also seem unlikely.
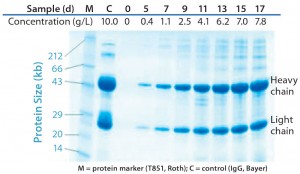
Figure 4: Quality of IgG1 produced during 17 days of high–cell-density fed-batch culture at 1,000-L scale assessd using sodium-dodecyl sulfate polyacrylamide gel electrophoresis (SDS PAGE) under reduced conditions and Coomassie blue staining; in addition to samples from the process start and days 5, 7, 9, 11, 13, 15, and 17 post-inoculum, the graph shows a marker (T851 from Roth) and control (IgG from Bayer).
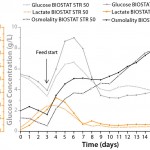
Figure 5: Glucose, lactate, and osmolality profiles of high–cell-density fed-batch processes in BIOSTAT STR 50 and BIOSTAT STR 1000 systems
Furthermore, we monitored glucose and lactate concentrations as well as osmolality during the fed-batch runs (Figure 5). Lactate concentration remained <2 g/L, below previously reported inhibitory levels (10). Glucose concentration was maintained at >3 g/L during the entire process. Osmolality ranged 300–470 mOsmol/kg and was kept below a previously established critical level of 550 mOsmol/kg (data not shown).
In essence, both our batch data and our fed-batch data are highly comparable across all investigated scales and in line with the results of our 5-L glass-vessel reference data. This demonstrates the superior biological performance of Flexsafe STR bags.
Successful Control of Critical Process Parameters
Minow et al. showed that detailed understanding of bioreactor performance enabled fast scale-up of a similar CHO fed-batch process (11). For our study, the extensive characterization of the BIOSTAT STR family with regard to oxygen transfer, mixing time, and power input summarized by de Wilde et al. (9) and the classical stirred-tank bioreactor design facilitated and simplified scale-up from 5 L to 1,000 L, as demonstrated by our highly comparable results.
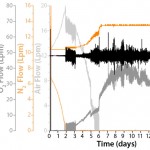
Figure 6: pO2 profile obtained in a high–cell-density fed-batch process
in a BIOSTAT STR 1000 system; in addition to pO2 control performance,
the graph shows stirrer rate, air flow, and nitrogen (N2) and pure oxygen
(O2) gas flow rates (cascade control strategy).
We ensured successful pO2 control at 60% ± 10% during the entire high– cell-density fed-batch process (Figure 6) using a multistage cascade-control approach based on pO2 signals from a single-use optochemical probe. Adding antifoam and changing from air to oxygen flow control caused minor variations in the pO2 value, especially at very low oxygen flow rates (Figure 6). We successfully controlled the pH value to 7.15 during the entire run (Figure 7) using signals from a single-use optochemical pH probe. During the fed-batch phase, daily supply of the highly alkaline Feed-B (pH 11) caused minor variations. For increased accuracy in pH control, we twice recalibrated the single-use probe signals (t = 3d, t = 6d ) based on off-line measurements (ΔpH > 0.05 units). The single-use and conventional glass pH probes demonstrated excellent comparability during the 1,000-L run, which we confirmed with off-line pH measurements. This demonstrated the capability of single-use sensors for stable control of pH and pO2 during a 17-day fed-batch production process.
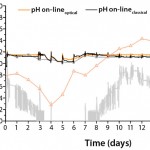
Figure 7: Comparing pH profiles of single-use optochemical probe (pH on-line optical) and conventional glass pH probe (pH on-line classical)
with off-line pH measurement (pH off-line) for a 1,000-L high–celldensity
fed-batch culture in the BIOSTAT STR 1000 system; further, CO2 gas flow rates and off-line values of pCO2
We monitored pCO2 values off-line using samples that were analyzed by an ABL800 Basic blood gas analyzer from Radiometer. Maximum values of 107 mmHg were reached on day 12 — well below a critical value of 150 mmHg reported by Zhu et al. (12). The pCO2 values obtained during our 1,000-L high–cell-density fed-batch run were comparable to data obtained in the BIOSTAT STR 50 fed-batch run (data not shown). Thus, successful CO2 stripping was achieved using the ring-sparger gassing strategy at the applied gas flow rates.
Conclusion
Our study successfully demonstrates the new Flexsafe STR and RM bag family’s suitability using a state of the art, high–cell-density, fed-batch MAb production process using CHO cells. We have confirmed data obtained from γ-irradiated sample bags during development of S80 polyethylene film (3) in the final bag assembly with a typical cell-culture application growing recombinant CHO cells in chemically defined, protein-free cell culture media. In light of recently published Dechema results (6), it becomes obvious how important optimizing polyethylene films for cell-culture applications is to ensuring reproducible growth performance. We hope to pave the way for full adoption of large-scale, single-use bioreactors in late-phase and commercial manufacturing, in which quality, reproducibility, and assurance of material supplies are critical for safe and uninterrupted drug availability.
Direct scale-up from a 5-L conventional glass stirred-tank vessel (BIOSTAT B 5), through a 50-L stirred-tank single-use bioreactor (BIOSTAT STR 50) to the final 1,000-L single-use stirred-tank system (BIOSTAT STR 1000) verified again the unique scalability of our entire BIOSTAT family, which is based on classical stirred-tank principle and single-use materials with superior growth-promoting properties. These results are also in line with previous studies that showed good agreement of the engineering design space and key process parameters of BIOSTAT stirred-tank bioreactors for mammalian cell culture (13). In addition to achieving the same cell culture performance across all investigated scales, we also demonstrated efficient control of key process parameters such as pH and pO2 in our very first high–cell-density fed-batch cultivation at 1,000-L scale.
In addition to similar design principles within the BIOSTAT stirred-tank bioreactor family, the combination of very effective CO2 stripping with high oxygen mass transfer provided by a ring sparger enabled our direct and successful scale-up. Finally, the performance of the optochemical pH and pO2 sensors demonstrates that a single-use bioreactor concept does not need to be compromised by insertion of classical probes that increase the risk of contamination. Our results convincingly show that a fully single-use cell culture process can be implemented easily and scaled reliably to production scale confirmed by excellent comparability of the 5-L, 50-L, and 1,000-L data.
Acknowledgments
Our thanks are due to the entire R&D upstream technology team for their technical contributions.
References
1 Eibl D, Peuker T, Eibl R. Single-Use Equipment in Biopharmaceutical Manufacture: A Brief Introduction. Single-Use Technology in Biopharmaceutical Manufacture. Eibl R, Eibl D., Eds. John Wiley and Sons: Hoboken, NJ, 2010.
2 Weber A, et al. Development and Qualification of a Scalable, Disposable Bioreactor for GMP-Compliant Cell Culture. BioProcess Int. 12(8) 2014: S47–S52.
3 Fenge C, et al. Consistently Superior Cell Growth Achieved with New Polyethylene Film Formulation. BioProcess Int. 12(8) 2014: S34–S37.
4 Vachette E, et al. Robust and Convenient Single-Use Processing with Superior Strength and Flexibility of Flexsafe Bags. BioProcess Int. 12(8) 2014: S38–S42.
5 Vachette E, et al. Enhanced Assurance of Supply for Single-Use Bags Based on Material Science, Quality By Design, and Partnership with Suppliers. BioProcess Int. 12(8) 2014: S43–S46.
6 Eibl R, et al. Recommendation for Leachable Studies: Standardized Cell Culture Test for the Early Identification of Critical Films for CHO Cell Lines in Chemically Defined Culture Media. Dechema January 2014.
7 Walsh G. Biopharmaceutical Benchmarks 2010. Nat. Biotechnol. 28(9) 2010.
8 Jurkiewicz E, et al. Verification of a New Biocompatible Single-Use Film Formulation with Optimized Additive Content for Multiple Bioprocess Applications. Biotechnol. Progr. in press.
9 De Wilde D, et al. Superior Scalability of Single-Use Bioreactors. BioProcess Int. 12(8)s 2014.
10 Zhang Y. Approaches to Optimizing Animal Cell Culture Process: Substrate Metabolism Regulation and Protein Expression Improvement. Adv. Biochem. Eng./Biotechnol. 113, 2009: 177–215.
11 Minow B, de Witt H, Knabben I. Fast Track API Manufacturing from Shake Flask to Production Scale Using a 1000-L Single-Use Facility. Chem. Ing. Technik 85(1–2) 2013: 87–94.
12 Zhu MM, et al. Effects of Elevated pCO2 and Osmolality on Growth of CHO Cells and Production of Antibody-Fusion Protein B1: A Case Study. Biotechnol. Progr. 21(1) 2005: 70–77.
13 Dreher T, et al. Design Space Definition for a Stirred Single-Use Bioreactor Family from 50 to 2000 L Scale. Eng. Life Sci. special issue (Single-Use Technologies in Biopharmaceutical Manufacturing). Eibl R, Eibl D, Eds. In press.
Corresponding author Regina Reglin and Sebastian Ruhl are scientists in upstream technology R&D; Jörg Weyand is a fermentation technologies application specialist in Central Europe; Davy De Wilde is director of marketing fermentation technologies; Ute Husemann is manager of upstream technology R&D; Gerhard Greller is R&D director of upstream technology; and Christel Fenge is vice president of marketing fermentation technologies at Sartorius Stedim Biotech, August-Spindler-Straβe 11, 37079 Goettingen, Germany; regina.reglin@ sartorius-stedim.com.