The acceptance and implementation of single-use systems (SUS) or “disposables” has increased strongly in bioprocess development and biopharmaceutical manufacturing over the past two decades. Typically, suppliers provide SUS presterilized and ready to use. Using SUS eliminates time-consuming and expensive cleaning procedures (which often require corrosive chemicals and a large amounts of water) and removes the need to perform cleaning validation between batches. The application of SUS reduces the risk of product cross-contamination and increases product and patient safety (1–5).
Polypropylene (PP) and polyethylene (PE) are common film-contact materials in disposable bags. Polycarbonate (PC) and cycloolefin copolymer (COC) are transparent polymers that can be used as alternatives to glass, and the elastomers ethylene-propylene-diene monomer rubber (EPDM) and methyl-vinyl-silicone rubber (MVQ) are used in seal applications or for pipes (6). Most single-use materials are polymers with limited material properties (1). To improve physiochemical properties, single-use–material developers include additives such as antioxidants, plasticizers, heat stabilizers, fillers, and colorants (7). Those additives and the extractable and leachable substances of polymers, however, can pose toxicity risks and reduce cell viability, therefore negatively influencing a cell culture process (8, 9).
Extractables are single compounds extracted from materials under exaggerated process conditions. A subset of extractables, leachables are compounds that migrate into process fluid during intended use (10). The presence of leachables in process fluids can lead to severe consequences. For example, in a case involving Eprex erythropoietin, a change in formulation caused leaching from uncoated rubber stoppers for syringes into the drug product. As a result, a significant number of patients developed an antibody-mediated pure red cell aplasia after a treatment with erythropoietin (11). Thus, extractables and leachables usually are discussed in risk assessments of SUS.
Evaluating extractables and leachables is an important aspect in quality by design (QbD) to ensure high product quality and safety. Such an assessment also helps biomanufacturers select single-use materials because explicit standardized tests for those materials do not exist (12–14). Instead, some companies adapt the international standards for the evaluation of medical devices (15). Below, we reference three types of qualitative in vitro cytotoxicity tests from ISO 10993-5 (tests for in vitro cytotoxicity) and US Pharmacopeia chapter <87> (biological reactivity, in vitro): extraction, direct contact, and indirect contact (agar diffusion/filter diffusion) tests (16–18). We chose the extraction test under standard cell-culture process conditions (cell culture medium and culture temperatures at 37 °C or 28 °C) because it most closely simulates the real application of SUS. Normally, cells should not grow directly on single-use materials (e.g., for vessel, tubes, and sensors) in single-use bioreactor processes.
The L929 murine fibroblasts cell line is recommended in both ISO and USP guidelines (16, 17), and it is respected as a reference cell line in our study. We also used a human embryonic kidney cell line (HEK293T) and the Spodoptera frugiperda 9 (Sf9) insect cell line because both are typically used as production cell lines in the biopharmaceutical industry (19, 20). All three cell types are continuous cell lines for in vitro cytotoxicity tests. That is because their biological responses are reproducible and because the use of primary cells is limited by their definite life spans and phenotypical changes after an increasing number of passages (21, 22).
The aim of our investigation was to test the influence of polymers that were extracted under process conditions and applied on cell lines commonly used in cell culture processes. We expected the results to differ from those of the study using reference cell line L929. We also adapted a test procedure for suspension cells, which typically are used in production processes, but are not considered in the guidelines.
We expanded the test setup and examined morphological changes and their influence on cell growth and viability. All three parameters are important in production processes. We also performed the 3-(4,5- dimethylthiazol-2-yl)-2,5-diphenyltetrazolium bromide (MTT) assay because of its orthogonal response. This colorimetric assay requires metabolically viable cells to convert MTT to formazan crystals (16, 23). Therefore, we did not assess morphology, but rather mitochondrial activity. For our third method, we used growth kinetics to obtain a cell growth parameter (e.g., doubling time as a cytotoxicity indicator). With these three different methods, we investigated the extracts of common, certified SUS polymers such as PP, PE, PC, COC, EPDM, and MVQ.
Material and Methods
Strain Maintenance: We purchased L929 cells from the Leibniz Institute German Collection of Microorganisms and Cell Cultures in Braunschweig (DSMZ no.: ACC 2). The cell line was cultured in RPMI 1640 media (from Bio&Sell) and supplemented with 10% (v/v) fetal bovine serum (FBS) and 2-mM L-glutamine (L-Gln) (both from Biochrom) in T-75 flasks (from Sarstedt). We maintained the cells at 37 °C in a humified 5% CO2 atmosphere. HEK293T cells (DSMZ no.: ACC 635) were grown in T-75 flasks (from Sarstedt) in Dulbecco’s modified Eagle’s medium (DMEM) (from Biochrom) and supplemented with 5% (v/v) FBS and 4-mM L-Gln at 37 °C in a humified 8% CO2 atmosphere.
We subcultured adherent cells twice per week. The cell layer was washed twice with phosphate-buffered saline (PBS) (from Sigma-Aldrich), dissociated with a trypsin-ethylenediaminetetra-acetic acid (EDTA) solution (from Biochrom), and resuspended in the culture medium. We determined cell concentration by using a 0.4% (w/v) trypan blue (from Sigma-Aldrich) dye-exclusion method and a Neubauer improved hemocytometer (from Paul Marienfeld GmbH & Co. KG). L929 and HEK293T cells were seeded at cell densities of 0.075 × 105 cells/cm2 and 0.37 × 105 cells/cm², respectively.
TriEx Sf9 cells (from Merck) were cultured in Sf-900 II serum-free media (SFM) (from Thermo Fisher Scientific) in 100-mL shake flasks (20-mL working volume) at 28 °C with 80 rpm on a Celltron shaker (from Infors HT) with a 25-mm throw. We subcultured cells twice per week to an initial cell concentration of 5 × 105 cells/mL. All cell lines were assayed periodically for mycoplasma.
Flow Cytometry with Propidium Iodide (PI) Staining: PI is a membrane-impermeant dye that penetrates damaged membranes of nonviable cells easily and thus can enable their exclusion from viable cells. Using this dye exclusion method, we determined cell viability using flow cytometry (Guava easyCyte benchtop flow cytometer from Merck Millipore). We followed a published protocol for measuring Sf9 cells (24).
Poly-d-Lysine Coating: To improve the attachment of HEK293T cells, we coated 96-well plates (from Sarstedt) with a ready-to-use poly-d-lysine (PDL, 0.1 mg/mL, from Thermo Fisher Scientific) solution. We prepared a 50-µg/mL working solution with sterile PBS (from Sigma-Aldrich), coated each well with 50 µL of the working solution, and incubated the solutions at room temperature for one hour. We removed PDL solution and rinsed the wells three times with 100 µL of sterile water. We dried the coated surface without lid under a laminar air flow for about two hours, then wrapped the plates with parafilm and stored them at 4 °C for up to two weeks after coating.
Positive Controls with Dimethyl Sulfoxide: We seeded adherent L929 cells at 0.05 × 105 cells/cm2 in 24-well plates. After 24 hours, we replaced the medium with a culture medium with 0.75% (v/v)–6% (v/v) dimethyl sulfoxide (DMSO from AppliChem). We carried out qualitative analysis and grading (Table 1) 48 hours after DMSO exposure using a Cytation 3 plate reader with Gen5 software (from BioTek Instruments).
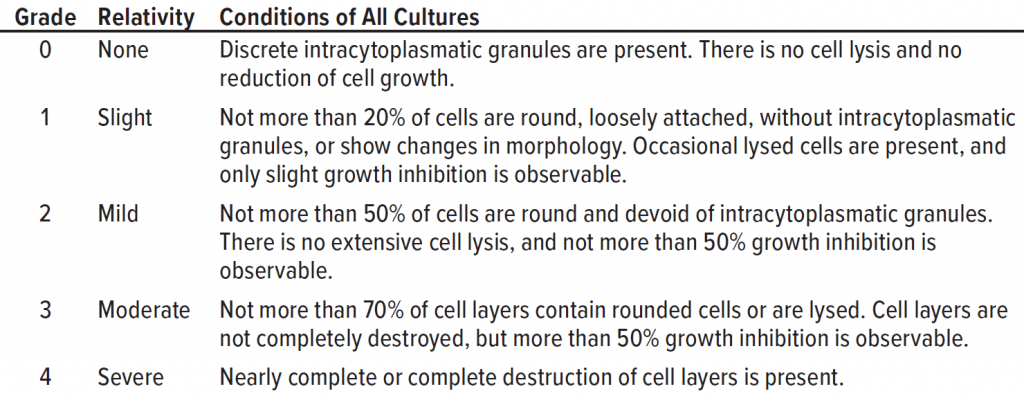
Table 1: Qualitative morphological grading of cytotoxicity of extracts is listed according to ISO 10993-5 (adapted from 16).
We seeded Sf9 cells at a cell concentration 5 × 105 cells/mL in six-well plates and in a culture medium with 0.75% (v/v)–6% (v/v) DMSO. We assessed viability every 24 hours, as described in the “flow cytometry” section above.
Extract Preparation of Test Material: We prepared extracts in accordance with ISO 10993-12 (25). All polymers were presterilized by gamma-irradiation with a dose of 52.3 kGy. In sterile glassware, thermoplastics (granulates) and the elastomers (o-rings) were weighed out with a mass-to-volume ratio of 0.2 g polymer per milliliter of extraction medium. We used a cell culture medium with added serum as an extraction vehicle. We added L-Gln when cells were treated with extracts.
We carried out extraction for seven days under the cultivation conditions of the specific cell line (adherent mammalian cell lines at 37 °C, insect cell line at 28 °C), imitating or exaggerating the cultivation time of a common cultivation. We used medium treated exactly as the extracts but without polymer interaction as a mock control. Fresh medium was a negative control. The 14-day mock control led to a slight growth inhibition with L929 cells compared with the results using the seven-day mock control and fresh medium. Extractions always were performed at 80–100 rpm, and all subsequent experiments were performed with undiluted extracts.
Starting Conditions for Qualitative Evaluation: For adherent cells, a subconfluent (~80% confluence) (16, 17), uniform, homogenously distributed cell layer after 24 hours is required. The confluence was determined with the phase contrast microscopy segmentation toolbox (PHANTAST) software tool (developed by University College London’s department of Biochemical Engineering and Complex and UCL Centre for Computation, Mathematics, and Physics) (26). The analysis enabled a more objective confluence analysis. We took photos at a 200-fold magnification and at defined coordinates on a plate reader. For an automated confluence analysis with the PHANTAST software, the threshold parameter ε was set to 0.1, and the scale parameter was set to 3. A seeding density of 0.5 × 105 cells/cm² for L929 cells and 0.75 × 105 cells/cm² for HEK293T cells were suitable to obtain the required subconfluence 24 hours after seeding.
Qualitative Morphological Grading: Adherent cells were seeded into a 96-well plate with 100 µL of culture medium. After 24 hours, a microscopic image of each well was taken in the plate reader at a 200-fold magnification. Then the culture medium was replaced with 100 µL of extract or controls. After 48 hours further of incubation, a second picture was taken, and the cells’ morphology, cell density, and confluence were assessed and graded in accordance with the definitions in Table 1. The classification or grading of cytotoxicity is defined in ISO 10993-5 and USP <87> and categorized into five grades from 0 to 4, depending on the cells’ morphology. A test sample meets regulation requirements if it is classified Grade 2 (mild reactivity) or lower (16, 17).
An MTT assay is used to compare the effect of extracts on metabolic activity (23). We carried out the assay as described in ISO 10993-5 Annex C (16). We dissolved MTT powder (from Sigma-Aldrich) in culture medium without phenol red (from Thermo Fisher Scientific) at a 1-mg/mL concentration. We sterile-filtrated the solution with a 0.22-µm filter (from Carl Roth GmbH) and seeded the cells with a volume of 100 µL at a cell density of 0.1 × 105 cells per well in a 96-well plate. For HEK293T cells, we used the PDL-coated surface as described above in the section about poly-d-lysine coating. Twenty-four hours after the cells were seeded, we removed the culture medium and added 100 µL of treated medium (extracts, mock control, and positive controls with DMSO). To exclude systematic errors, such as incorrect seeding, we examined each plate with an incident-light microscope (from Leica Camera) and incubated cells a second time. We removed the medium after 24 hours and added 50 µL of MTT solution per well. The plate was then incubated for two hours at cultivation conditions; then the MTT solution was discarded. We added 100 µL isopropanol (from Carl Roth) to the plate wells and agitated the plate for 30 minutes at 100 rpm on a Celltron shaker. Subsequently, we measured the absorbance at 570 nm (reference wavelength 650 nm) for the samples in the plate reader. Absorption of dissolved formazan correlates with the number of living cells with metabolic activity (23). We compared all samples, including mock and positive controls, with the negative control and used Equation 1 to calculate cell viability.
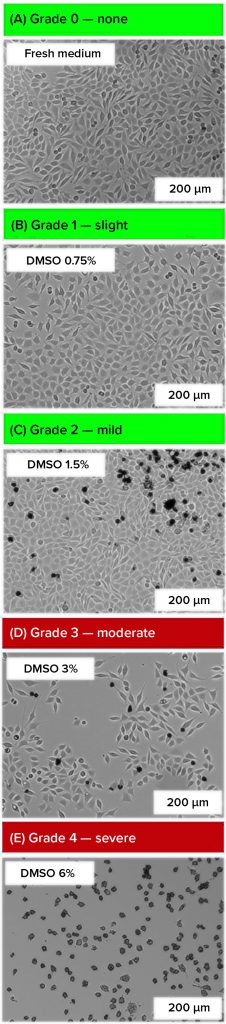
Figure 1 (a-e): Microphotographs were taken 48 hours after addition of dimethylsulfoxide (DMSO) (adherent L929 cells seeded at 0.05 × 105 cells/cm², 200-fold magnification). Grading complies with ISO 10993-5 and USP <87>, adjusted by the addition of different concentrations of DMSO in the cell culture medium.
We performed growth kinetics with HEK293T and Sf9 cells. The adherent HEK293T cells were seeded at a density of 0.15 × 105 cells/cm2 in six-well plates with a working volume of 3 mL per well, and in BioCoat 24-well plates (from Corning) with a working volume of 2 mL per well. Twenty-four hours after seeding, we replaced the medium with extracts and controls. Every 24 hours, we measured glucose and lactate concentration of the supernatant with Biosen C-Line GP+ (from EKF Diagnostics) and the cell concentration. For that, cells were washed twice with PBS before detachment with 300 µL (six-well) or 100 µL (24-well) trypsin-EDTA solution per well. We stopped the detachment reaction with a serum-containing culture medium and counted the cells. We seeded the insect cells in extracts or controls at a seeding concentration of 5 × 105 cells/mL in six-well plates with a working volume of 2.5 mL per well. A change of medium including centrifugation could damage the cells. Well plates were agitated at 80 rpm on a Celltron shaker. Every 24 hours, we measured the cell concentration and the glucose and lactate concentration.
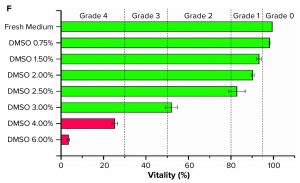
Figure 1 (f): Graph shows grading of suspended Sf9 cells (seeded at 5 × 105 cells/mL, n = 3) with different DMSO concentrations in their culture medium.
Results
Qualitative Morphological Grading with Adherent Cell Lines, and Grading with Suspension Cell Line: With the L929 cell line, the five grades of cytotoxic influence (Table 1) could be replicated by using different concentrations of the cytotoxic substance DMSO (Figure 1 a–e) (27, 28). Usually, L929 cells are adherent cells with a spindle-shaped morphology, growing as a confluent monolayer. Cells that were cultivated in a fresh medium showed a typical morphology (Grade 0) and no reduction in growth (Figure 1a). After using a DMSO concentration of 0.75% (v/v), we observed a slight growth inhibition, characterized by a less dense cell layer (Grade 1) (Figure 1b). After using 1.5% (v/v) DMSO, a few detached, round cells were visible on the cell layer (Grade 2) (Figure 1c). Using concentrations of 3% (v/v) DMSO led to a strong growth inhibition, but the cell layer was not completely destroyed (Grade 3) (Figure 1d). After using 6% (v/v) DMSO, we observed a complete destruction of cells (Grade 4) (Figure 1e).
For suspension cells, we adapted the morphology grading. We established a classification for Sf9 cells by a live–dead staining with propidium iodide. The cytotoxic influence of different DMSO concentrations led to different viabilities after incubation and was matched with the five grades (Figure 1f). We determined the viability grading 96 hours after seeding to eliminate the impact of the adaption phase because the viability of the control group first dropped and then recovered again by 100%.
Only the Sf9 cell samples with 6% (v/v) DMSO could be distinguished from other samples when we observed them with a microscope. Most of the cells were lysed. The viability was lower than 5% (Grade 4). Using a concentration of 4% (v/v) and 3% (v/v) DMSO, viability after 96 hours was ~25% (Grade 4) and just above 50%, respectively (Grade 2). Using DMSO concentrations of 2.5% (v/v) to 1.5% (v/v) resulted in a viability of ~80% to ~90% (Grade 1). Using DMSO concentrations <0.75% (v/v) had no negative impact on viability (Grade 0).
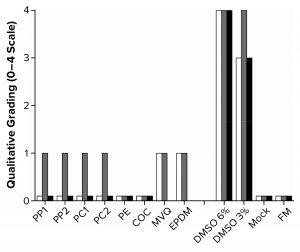
Figure 2: Graph shows qualitative morphological grading of the polymer extracts. White bars are L929 cells (seeded at 0.5 × 105 cells/cm with 48 hours incubation in a 96-well plate, n = 8); gray bars are HEK293T cells (seeded at 0.75 × 105 cells/cm with 48 hours incubation in a 96-well plate, n = 8); black bars are Sf9 cells (seeded at 5 × 105 cells/mL with 96 hours incubation in a six-well plate, n = 3), with no results for MVQ and EPDM. FM = fresh medium.
With those methods, we determined the cytotoxicity grades of all polymers on the three cell lines (Figure 2). In the cases of PP, PC, MVQ, and EPDM, a slight growth inhibition was observed with HEK293T cells (Grade 1), whereas L929 cells showed a slightly less dense cell layer after incubation with MVQ and EPDM extracts (Grade 1) but not for the PP and PC extracts (Grade 0). The polymers PE and COC did not negatively influence the cell lines (Grade 0). The HEK293T cells reacted more sensitively toward positive controls with 3% (v/v) DMSO (Grade 4) than the L929 cells did (Grade 3).
All polymer extracts tested on Sf9 cells could be graded with Grade 0. Despite the influence of polymer extracts, viabilities of 100% after 96 hours were achieved with the mock control and fresh medium alike.
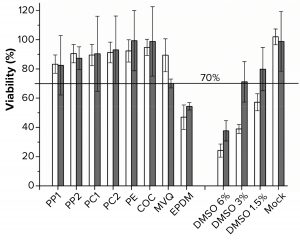
Figure 3: Viability was determined by using the MTT assay. White bars are L929 cells (n = 5), gray bars are HEK293T cells (n = 10). Polymers used for extraction were polypropylene (PP), polycarbonate (PC), polyethylene (PE), cyclic olefin copolymer (COC), methyl-vinyl silicone (MVQ), ethylene propylene diene monomer (EPDM) rubber elastomers, controls of 6%, 3%, 1.5% dimethylsulfoxide (DMSO), and mock for both cell lines.
MTT Assay with L929 and HEK293T Cells: Although the polymer extracts do not kill the cells, they could compromise their metabolic activity (16, 23). We performed the MTT assay as an orthogonal method to determine cell viability over metabolic activity. For the MTT assay, ISO 10993-5 defines a cell viability <70% as an indicator for a cytotoxic potential (16). Because of the test methodology, we applied the MTT test to only adherent cells (Figure 3). PP, PC, PE, and COC extracts did not lead to a cell viability lower than the critical level of 70%.
The viability of cells cultivated in the PP1 extract was the lowest, at just above 80%. For the PE and COC extracts, a ~100% viability of HEK293T cells was achieved. Viability dropped to ~50% in both cell lines after incubation in an EPDM extract. By contrast, the other elastomer extract MVQ led to viabilities of 70% (HEK293T cells) and 90% (L929 cells), respectively. The mock control showed no significant decrease in viability. As we expected, the positive controls had a DMSO concentration-dependent decrease of viability.
Growth Kinetics with HEK293T and Sf9 Cells: Cytotoxic substances do not necessarily have to reduce metabolic activity to negatively affect a culture. A reduced growth or a lowered final cell concentration also can indicate cytotoxicity (9, 16). Another method for quantitative evaluation is to perform growth kinetics, which we did for HEK293T and Sf9 cells (Table 2). Small polymer particles were visible in the PP extracts, especially in the PP1 extract. We tested both PP extracts with the particles, and after we filtrated the samples with a 0.22-µm syringe filter, we labeled them with an asterisk (*).
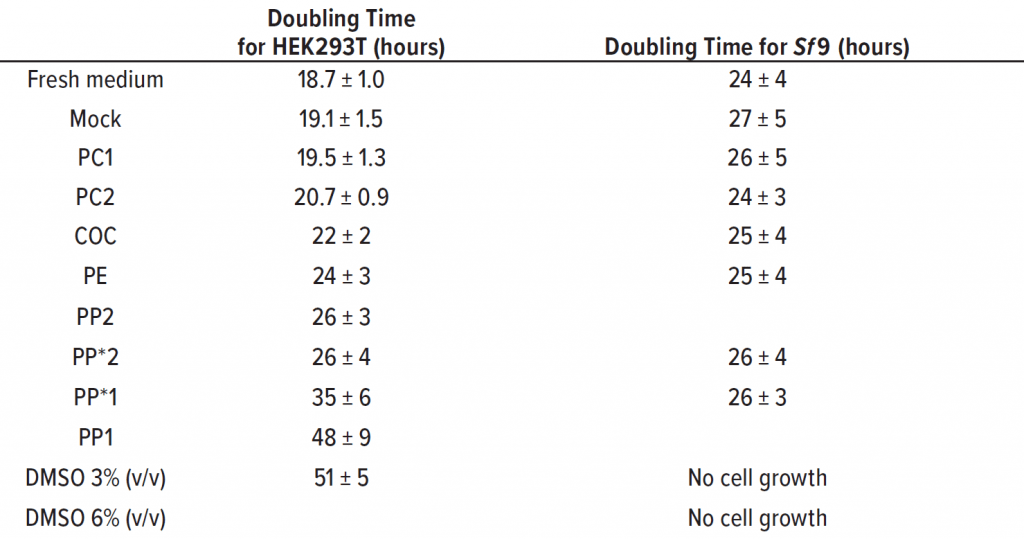
Table 2: Doubling time was calculated from growth rates (24–120 hours) for HEK293T (seeding density 0.15 × 105 cells/cm2) and Sf9 (seeding concentration 5 × 105 cells/mL) (n = 3). Samples filtrated with 0.22-μm syringe filter are labeled with an asterisk (see text).
The PP1 and PP*1 samples showed round HEK293T cells without adherence to the growth surface. Most cells conglomerated in the middle of the culture vessel. Those observations are reflected in a slow growth with an increased doubling time of 48 ± 9 h in PP1 extract, compared with the doubling time in fresh medium of 18.7 ± 1.0 h. Thus, there was no significant deviation between the growth in PP1 extract and the positive control with 3% (v/v) DMSO over 51 ± 5 h.
Through a filtration of PP, extracts should prove that the polymer particles are not causal for the cytotoxic effect of the PP1 extract. The filtrated extract PP*1 also inhibited cell growth (doubling time of 35 ± 6 h), indicating cytotoxic leachable compounds in the extract of PP*1. The cell-specific glucose uptake and lactate production rates were doubled under the influence of PP1 and PP*1 extracts compared with the negative control.
Despite the presence of particles in the PP2 samples, most of those cells were adherent and showed an inconspicuous morphology. The doubling times of filtrated and untreated extracts were similar at ~26 h, but higher than for the negative control. Also, the cell-specific glucose uptake and lactate production rates were increased (~1.5-fold) under the influence of PP2/PP*2 extracts.
Our polypropylene study also showed that the same materials from different manufacturers can lead to a difference in cytotoxicity. Table 2 shows that the doubling times of HEK293T cells that were treated with PE and COC extracts (~24 h and ~22 h, respectively) were slightly greater than the doubling time of the negative control. Doubling time of HEK293T cells that were treated with PC extracts was comparable with that of the mock control, and no significant deviations could be observed. The cell-specific glucose uptake and lactate production rates also were comparable with those of the mock control and fresh medium control. Cells under DMSO influence showed threefold increases in specific glucose uptake and lactate production rates.
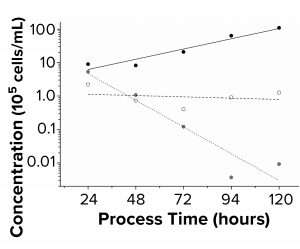
Figure 4: Sf9 growth kinetics (n = 3) were
charted. Black symbols are fresh medium;
white symbols are DMSO 3% (v/v); gray
symbols are DMSO 6% (v/v). Seeding
concentration was 5 × 105 cells/mL in a sixwell
plate.
As Table 2 shows, doubling time for all tested polymer extracts with Sf9 cells were comparable with the 24 ± 4 h doubling time of the fresh medium control. PP extracts were both filtrated to eliminate particles during flow cytometry. Figure 4 shows that the positive controls with 3% (v/v) and 6% (v/v) DMSO led to cell death. The formation of lactate was below the limit of detection of 0.05 g/L, and the cell-specific glucose uptake rates under the influence of polymer extracts were comparable with those of the controls.
Discussion
This work focused on the assessment of test procedures for the in vitro cytotoxicity of polymers and other materials for SUS. We adapted the ISO 10993-5 and USP <87> guidelines for medical devices to analyze the in vitro cytotoxicity of SUS polymers. Those sources also provide some information about the formation of extractables and leachables under cell culture process conditions.
Impact of Extraction Procedure: The extraction medium, extraction time, and temperature are important factors of an extraction and leaching process study. Because we wanted to best simulate real process conditions for the extractables testing, we used cell culture medium for the extraction so that the extracts could be tested without their dilution or modification. A culture medium with a serum is especially suitable for such experiments because it extracts polar and nonpolar substances and supports cell growth. Thus, a serum-containing culture medium is preferred over water as an extraction vehicle (16, 17, 25, 29).
To preclude a temperature-induced degradation of media components, we carried out the extraction at a temperatures equal to the usual cultivation temperatures of 28 °C and 37 °C (30–32). We tested an extraction time longer than required in ISO 10993-5 and USP <87>, but we observed that cells cultivated in the medium with a 14-day extraction time and a mock control showed inhibited growth. Thus, the longer incubation time had a negative effect on medium supplements, and a 14-day extraction went beyond the objective (30–32). We also found an incubation time of seven days to be suitable and recognized no difference between mock control and cells cultivated with fresh medium. We strongly recommend the use of mock and negative controls to verify that observed effects are induced by the extracts and not by another environmental factor.
Impact of Detection Method: Extractables and leachables can be cytostatic, destroy cell compartments, and affect specific cell functions that are not visible by means of qualitative evaluation (33). Thus, the choice detection method is crucial. An evaluation with a focus on morphological abnormalities is applicable mainly for adherent cells. Visible grading is a useful method for a first cytotoxicity assessment. The procedure is simple and requires no specialized equipment or chemicals. The main disadvantage of this method is experimenter subjectivity. By reproducing the grades with different DMSO concentrations (Figure 1a–e) before the main tests, we enabled a more consistent comparison. Although the ISO 10993-5 refers to qualitative grading as appropriate for screening purposes, we and others recommend addressing the difficulty of subjectivity of the qualitative evaluation, which should be controlled against a quantitative assessment (16, 25).
The MTT assay is a well-established quantitative method. Herein, several parameters such as the influence of serum and phenol red and the effectivity of a lysing agent have been discussed (34–36). Although different procedures are available for this study, ours is based on the protocol of ISO 10993 5. The removal of extracts ensures that extractables or medium compounds cannot react with the MTT assay. The main drawback of that approach is that discarding the MTT before adding the solving agent also can remove formazan crystals, thus affecting results. Because of the frequent medium removal stated in the ISO 10993-5 protocol, the attachment of HEK293T cells was not strong enough in our study. We removed cells by changing the medium, which led to false results. We reached an increased adherence with a PDL-coated surface for HEK293T cells (37). Our second quantitative approach was an investigation of growth kinetics to evaluate parameters such as the inhibition of cell growth, substrate consumption, and the formation of metabolic metabolites.
We believe that the MTT assay and the growth kinetics evaluation are useful methods for identifying materials. They do not change cell morphology but instead influence the metabolism of cells or their growth. Thus, they are orthogonal methods for classification of materials.
Adaption of Test Procedure for Suspension Cells: Suspension cell culture is a common platform in biopharmaceutical production. Industry guidelines, however, do not provide a proposal for grading of suspension cells. Grading based on changes in cell morphology is impractical, so qualitative grading recommended as the method of choice in ISO 10993 5 and USP <87> is not applicable.
In our study, we used flow cytometry and live–dead staining with PI to determine cell viability and enable a classification for suspension cells such as Sf9 cells. The proposed grading method follows the attribution of grades in accordance with ISO 10993-5 and USP <87>. After defining the grades described in the official requirements with the cytotoxic solvent DMSO (Figure 1f), we developed a method for comparing suspension cells such as the Sf9 insect cell line. Table 1 lists the definition of those grades. Nevertheless, note that the reproduction of the grades with a cytotoxic substance (e.g., DMSO) must be performed before testing extractables and leachables every time a different suspension cell line is to be tested. That is because each cell line reacts differently to the cytotoxic substance in different concentrations. Provided that the grades can be reproduced with a cytotoxic substance, our approach enables the identification of polymer extractables and leachables that reduce cell viability. For the MTT assay, modified procedures (without the removal of the medium and another composition of dissolving solution) applied to suspension cells are described in literature (38).
Different Outcomes with Different Detection Methods: No grading of 2 or higher was observed in the qualitative morphological analysis for all polymers (PP, PC, PE, COC, MVQ, and EPDM) and both adherent cell lines (Table 3). That indicates that all materials are suitable to produce SUS. A second evaluation after conducting the orthogonal method of the MTT assay revealed that MVQ and EPDM did not influence the cell layer significantly enough to be graded 2 or higher, but they reduced metabolic activity significantly when we compared metabolic activity results of the other polymer extracts and controls.
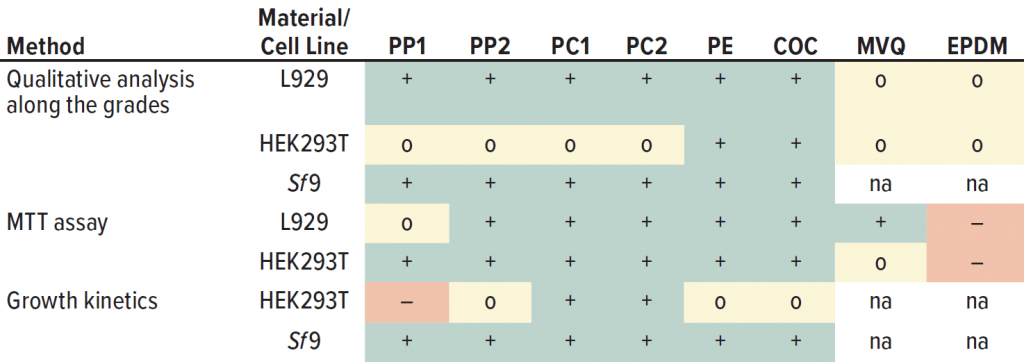
Table 3: Summarized results from all cytotoxicity tests for all methods and cell lines used; – = cytotoxic effect; o = slight noncritical effect; + = no negative effect, na = not available.
For the MVQ extract, the limit for a “cytotoxic” classification of 70% viability was just reached for HEK293T cells, whereas for EPDM a cytotoxic effect was clearly observed (Table 3). That detrimental effect of the material EPDM (e.g., used for o-rings) already has been described for single-use applications to HEK cells (39), whereas other studies confirmed biocompatibility of EPDM (40). Thus, materials must be evaluated case by case before their application in a cell culture process. Our growth kinetics revealed that PP negatively influenced the growth of adherent-growing HEK293T cells. Results of the qualitative analysis and the MTT assay were inconspicuous. Other studies have reported that adherent cells showed a decreased glucose consumption and lactate formation as a consequence of cytotoxic ZnO nanoparticles, which are used as activators in the plastics industry (41). Our results showed a decreased glucose consumption and lactate formation because of PP extracts and positive controls, consistent with the increased metabolic cell specific rates. That indicates a cytotoxic influence.
Our results showed no significant inhibition of cell growth and mitochondrial activity (MTT assay) for PE and PC extracts. Other studies have reported poor cell growth in single-use bags with PE as a fluid-contact material — a result that was caused by the breakdown product bis(2,4-di-tert-butylphenyl)phosphate (bDtBPP) of an antioxidant additive that migrated into the process fluid (9, 42). A leaching of the cell growth inhibitory compound 3,5-dinitro-bisphenol A also has been reported for PC equipment (43). Because the polymer compositions, including the additives, differ among manufacturers, the evaluation of one polymer from one manufacturer with one certain medium is not transferable to all media and all manufacturers.
Different Outcomes with Different Cell Lines: In all three methods, our results showed differences across the three cell lines we investigated. The L929 cells were most sensitive in the MTT assay but inconspicuous in the morphological grading. The HEK293T cells were sensitive in the morphological grading and growth kinetics, but those cells were more robust in the MTT assay.
Sf9 cells were highly robust in morphological grading, and they had no negative influence on growth for all polymers tested (Table 3). Sf9 cells treated with polymer extracts reached the same doubling times (24 ± 4 h) as did the untreated control cells (Table 2). Other studies have reported that doubling times vary between 24 and 31 hours (44). We did not detected lactate formation, which could indicate that the insect cells were stressed (45, 46). Thus, potential leachables had no influence on the growth of Sf9 cells. But we note that the extraction temperature was 28 °C instead of 37 °C. The release of leachables increases at high extraction temperatures (42).
Compared with Sf9 insect cells, adherent HEK293T cells seemed to react more sensitively to the polymer extracts. A similar relation was observed for a sensitivity test with Sf9 cells and Chinese hamster ovary (CHO) cells on leachable compounds of single-use bags (47). Those results further solidify our finding that using one method or cell line for categorizing a material as “compatible” or “cytotoxic” does not necessarily lead to a solid result. That is in agreement with other studies reporting different cell-line–dependent biological responses to toxic substances (48–50).
Limits of the Cytotoxicity Determination: In vitro cytotoxicity testing measures the cytotoxic effect of a material without specifying the cause. The tests cannot explain the reasons for an occurred cytotoxicity. But besides the established extractables and leachables studies, which identify specific components, the benefit of cell-culture–specific tests for an estimation of the effect on production cell lines is without doubt. Standardized cell culture tests would improve the quality of single-use equipment (13, 14), but we recommend adopting those tests case by case, best mimicking exact process conditions. Altogether, it becomes apparent that no single method conducted on one specific cell line is sufficient for the categorization of a material as being biocompatible or cytotoxic. An orthogonal approach is a more sophisticated way to determine whether a material is suitable for a certain cell line in a certain process. The same material could lead to different assessments in different tests and a more realistic determination of the cytotoxicity of a material can be made by testing with a production cell line.
Orthogonal Testing Is Key
Raw materials for SUS must be examined for extractables and leachables formation and their potential detrimental effect on producing cells and on product quality and safety. Concluding all results of the different cytotoxicity studies described herein, even extracts of the same polymer acquired from different manufacturers lead to different outcomes in different cytotoxicity tests. Our findings demonstrate the necessity of cytotoxicity testing to be performed on a cell line, a material, and a manufacturing process on a case-by-case basis. Even polymers commonly used for SUS in cell cultures can influence growth and viability in a specific process. Results will depend on the extraction procedure, the choice of cytotoxicity detection method (better using several methods with orthogonal test principles instead of only one), the sensitivity of a production cell line, and so on. We recommend that if a material component is exchanged for another in a SUS, then its safety assessment should be reviewed. Because we obtained different results from different testing methods, we conclude that using at least two orthogonal testing methods to classify polymeric materials is a reasonable approach to reduce uncertainty.
Acknowledgments
This work was funded by the German Federal Ministry of Economic Affairs and Energy (BMWi, project acronym: OptoBag, grant number: 4783502BA9). We thank the Hessen State Ministry of Higher Education, Research, and the Arts for the financial support within the Hessen initiative for scientific and economic excellence (LOEWE Program), the LOEWE Center for Insect Biotechnology and Bioresources (ZIB), and the LOEWE Center Novel Drug Targets Against Poverty-Related and Neglected Tropical Infectious Diseases initiative (DRUID). The authors acknowledge Catherine Meckel-Oschmann for editing the manuscript.
References
1 Eibl D, Eibl R. Single-Use Equipment in Biopharmaceutical Manufacture. Single-Use Technology in Biopharmaceutical Manufacture. Eibl R, Eibl D, Eds. John Wiley & Sons: Hoboken, NJ, 2019; 1–11; https://doi.org/10.1002/9781119477891.ch1.
2 Eibl D, Eibl R, Köhler P. Single-Use Technology in Biopharmaceutical Production: Report of the Temporary Working Group. Dechema Biotechnologie: Frankfurt, Germany, 2012; https://dechema.de/-p-20055310/_/StatPap_SingleUse_2011_englisch.pdf.
3 Lopes AG. Single-Use in the Biopharmaceutical Industry: A Review of Current Technology Impact, Challenges, and Limitations. Food Bioprod. Process. 93, 2015: 98–114; https://doi.org/10.1016/j.fbp.2013.12.002.
4 Shukla AA, Gottschalk U. Single-Use Disposable Technologies for Biopharmaceutical Manufacturing. Trends Biotechnol. 31(3) 2013: 147–54; https://doi.org/10.1016/j.tibtech.2012.10.004.
5 Bayer M, et al. Technical State-of-the-Art and Risk Analysis on Single-Use Equipment in Continuous Processing Steps: Expert Group Single-Use Technology. Dechema Biotechnologie: Frankfurt, Germany, 2020; https://www.sartorius.com/download/499818/2020-single-use-continuous-processes-2020-ezl-p-20006074-data.pdf.
6 Vanhamel S, Piton C. Production of Disposable Bags. Single-Use Technology in Biopharmaceutical Manufacture. Eibl R, Eibl D, Eds. John Wiley & Sons: Hoboken, NJ, 2019; 95–116; https://doi.org/10.1002/9781119477891.ch8.
7 Hahladakis JN, et al. An Overview of Chemical Additives Present in Plastics: Migration, Release, Fate, and Environmental Impact During Their Use, Disposal, and Recycling. J. Hazard. Mater. 344, 2018: 179–99; https://doi.org/10.1016/j.jhazmat.2017.10.014.
8 Cuadros-Rodríguez L, et al. Leachables from Plastic Materials in Contact with Drugs. State of the Art and Review of Current Analytical Approaches. Int. J. Pharm. 583, 2020: 119332; https://doi.org/10.1016/j.jhazmat.2017.10.014.
9 Hammond M, et al. A Cytotoxic Leachable Compound from Single-Use Bioprocess Equipment That Causes Poor Cell Growth Performance. Biotechnol. Prog. 30(2) 2014: 332–337; https://doi.org/10.1002/btpr.1869.
10 Jenke D. Evaluation of the Chemical Compatibility of Plastic Contact Materials and Pharmaceutical Products: Safety Considerations Related to Extractables and Leachables. J. Pharm. Sci. 96(10) 2007: 2566–2581; https://doi.org/10.1002/jps.20984.
11 McKoy JM, et al. Epoetin-Associated Pure Red Cell Aplasia: Past, Present, and Future Considerations. Transfusion 48(8) 2008: 1754–1762; https://doi.org/10.1111/j.1537-2995.2008.01749.x.
12 Tappe A, et al. The Case for a Standardized Assay to Test Suitability of Single-Use Systems in Cell Culture Applications. BioProcess Int. 14(1) 2016: 10–13; https://lne.e92.mwp.accessdomain.com/upstream-processing/assays/the-case-for-a-standardized-assay-to-test-suitability-of-single-use-systems-in-cell-culture-applications.
13 Eibl R, et al. Recommendation for Leachables Studies: Standardized Cell Culture Test for the Early Identification of Critical Films. 1st ed. Dechema Biotechnologie: Frankfurt, Germany, 2014.
14 Wood J, Mahajan E, Shiratori M. Strategy for Selecting Disposable Bags for Cell Culture Media Applications Based on a Root-Cause Investigation. Biotechnol. Prog. 29(6) 2013: 1535–1549; https://doi.org/10.1002/btpr.1802.
15 ISO 10993-1:2009: Biological Evaluation of Medical Devices, Part 1 —Evaluation and Testing Within a Risk Management Process. International Organization for Standardization: Geneva, Switzerland; https://www.iso.org/standard/44908.html.
16 ISO 10993-5:2009: Biological Evaluation of Medical Devices, Part 5 — Tests for In Vitro Cytotoxicity. International Organization for Standardization; Geneva, Switzerland; https://www.iso.org/standard/36406.html.
17 <87> Biological Reactivity Tests, In Vitro. USP43–NF38. The United States Pharmacopeial Convention, Rockville, MD.
18 Liu X, et al. A Comparison of In Vitro Cytotoxicity Assays in Medical Device Regulatory Studies. Regul. Toxicol. Pharmacol. 97, 2018: 24–32; https://doi.org/10.1016/j.yrtph.2018.06.003.
19 Weber W, Fussenegger M. Insect Cell-Based Recombinant Protein Production. Cell and Tissue Reaction Engineering. Eibl R, et al., Eds. Springer: Berlin–Heidelberg, Germany, 2009: 263–277; https://doi.org/10.1007/978-3-540-68182-3_6.
20 Dumont J, et al. Human Cell Lines for Biopharmaceutical Manufacturing: History, Status, and Future Perspectives. Crit. Rev. Biotechnol. 36(6) 2016: 1110–1122; https://doi.org/10.3109/07388551.2015.1084266.
21 Thonemann B, et al. Responses of L929 Mouse Fibroblasts, Primary and Immortalized Bovine Dental Papilla-Derived Cell Lines to Dental Resin Components. Dent. Mater. 18(4) 2002: 318–323; https://doi.org/10.1016/s0109-5641(01)00056-2.
22 Schedle A, et al. Response of L929 Fibroblasts, Human Gingival Fibroblasts, and Human Tissue Mast Cells to Various Metal Cations. J. Dent. Res. 74(8) 1995: 1513–1520; https://doi.org/10.1177/00220345950740081301.
23 Mosmann T. Rapid Colorimetric Assay for Cellular Growth and Survival: Application to Proliferation and Cytotoxicity Assays. J. Immunol. Methods 65(1–2) 1983: 55–63. https://doi.org./10.1016/0022-1759(83)90303-4.
24 Harnischfeger J, et al. Bioreactor-Based Antigen Production Process Using the Baculovirus Expression Vector System. Methods Mol. Biol. 2183, 2021: 95–118; https://doi.org/10.1007/978-1-0716-0795-4_8.
25 ISO 10993-12:2021: Biological Evaluation of Medical Devices, Part 12 — Sample Preparation and Reference Materials. International Organization for Standardization: Geneva, Switzerland; https://www.iso.org/standard/75769.html.
26 Jaccard N, et al. Automated Method for the Rapid and Precise Estimation of Adherent Cell Culture Characteristics from Phase Contrast Microscopy Images. Biotechnol. Bioeng. 111, 2014: 504–517; https://doi.org/10.1002/bit.25115.
27 Gurtovenko AA, Anwar J. Modulating the Structure and Properties of Cell Membranes: The Molecular Mechanism of Action of Dimethyl Sulfoxide. J. Phys. Chem. B. 111(35) 2007: 10453–10460; https://doi.org/10.1021/jp073113e.
28 Santos NC, et al. Multidisciplinary Utilization of Dimethyl Sulfoxide: Pharmacological, Cellular, and Molecular Aspects. Biochem. Pharmacol. 65(7) 2003: 1035–1041; https://doi.org/10.1016/S0006-2952(03)00002-9.
29 Baek HS, et al. Evaluation of the Extraction Method for the Cytotoxicity Testing of Latex Gloves. Yonsei Med. J. 46(4) 2005: 579–583; https://doi.org/10.3349/ymj.2005.46.4.579.
30 Jagušić M, et al. Stability of Minimum Essential Medium Functionality Despite l-Glutamine Decomposition. Cytotechnol. 68(4) 2016: 1171–1183; https://doi.org/10.1007/s10616-015-9875-8.
31 Khan K, Elia M. Factors Affecting the Stability of l-Glutamine in Solution. Clin. Nutr. 10(4) 1991: 186–192; https://doi.org/10.1016/0261-5614(91)90037-D.
32 Schnellbaecher A, et al. Vitamins in Cell Culture Media: Stability and Stabilization Strategies. Biotechnol. Bioeng. 116(6) 2019: 1537–1555; https://doi.org/10.1002/bit.26942.
33 Ciapetti G, et al. In Vitro Evaluation of Cell/Biomaterial Interaction By MTT Assay. Biomaterials 14(5) 1993: 359–364; https://doi.org/10.1016/0142-9612(93)90055-7.
34 Tada H, et al. An Improved Colorimetric Assay for Interleukin 2. J. Immunol. Methods 93(2) 1986: 157–165; https://doi.org/10.1016/0022-1759(86)90183-3.
35 Denizot F, Lang R. Rapid Colorimetric Assay for Cell Growth and Survival. J. Immunol. Methods. 89(2) 1986: 271–277; https://doi.org/10.1016/0022-1759(86)90368-6.
36 Hansen MB, Nielsen SE, Berg K. Reexamination and Further Development of a Precise and Rapid Dye Method for Measuring Cell Growth/Cell Kill. J. Immunol.Methods. 19(2) 1989: 203–210; https://doi.org/ 10.1016/0022-1759(89)90397-9.
37 Vancha AR, et al. Use of Polyethyleneimine Polymer in Cell Culture as Attachment Factor and Lipofection Enhancer. BMC Biotechnol. 4, 2004: 23; https://doi.org/10.1186/1472-6750-4-23.
38 Nga NTH, et al. Optimization and Application of MTT Assay in Determining Density of Suspension Cells. Anal. Biochem. 610, 2020: 113937; https://doi.org/10.1016/j.ab.2020.113937.
39 Aizawa P, et al. Effect of Different Materials Used in Bioreactor Equipments on Cell Growth of Human Embryonic Kidney (HEK293) Cells Cultivated in a Protein-Free Medium. Cell Technology for Cell Products: Proceedings of the 19th ESACT Meeting. Smith R, Ed. Springer: Dordrecht, the Netherlands, 2007: 459–462; https://doi.org/10.1007/978-1-4020-5476-1_77.
40 Mast F, et al. In Vitro Biocompatibility of EPM and EPDM Rubbers. J. Mater. Sci. Mater. Med. 8(1) 1997: 5–9; https://doi.org/ 10.1023/a:1018577910511.
41 Lai X, et al. The Effect of Fe2O3 and ZnO Nanoparticles on Cytotoxicity and Glucose Metabolism in Lung Epithelial Cells. J. Appl. Toxicol. 35(6) 2015: 651–664; https://doi.org/10.1002/jat.3128.
42 Hammond M, et al. Identification of a Leachable Compound Detrimental to Cell Growth in Single-Use Bioprocess Containers. PDA J. Pharm. Sci. Technol. 67(2) 2013: 123–134; https://doi.org/10.5731/pdajpst.2013.00905.
43 Peng J, et al. Chemical Identity and Mechanism of Action and Formation of a Cell Growth Inhibitory Compound from Polycarbonate Flasks. Anal Chem. 90(7) 2018: 4603–4610; https://doi.org/10.1021/acs.analchem.7b05102.
44 Wang P, Granados RR, Shuler ML. Studies on Serum-Free Culture of Insect Cells for Virus Propagation and Recombinant Protein Production. J. Invertebrate Pathol. 59(1) 1992: 46–53; https://doi.org/10.1016/0022-2011(92)90110-P.
45 Drews M, Paalme T, Vilu R. The Growth and Nutrient Utilization of the Insect Cell Line Spodoptera frugiperda Sf9 in Batch and Continuous Culture. J. Biotechnol. 40(3) 1995: 187–198; https://doi.org/10.1016/0168-1656(95)00045-R.
46 Ikonomou L, Schneider Y-J, Agathos SN. Insect Cell Culture for Industrial Production of Recombinant Proteins. Appl. Microbiol. Biotechnol. 62, 2003: 1–20; https://doi.org/10.1007/s00253-003-1223-9.
47 Blaschczok K, et al. Evaluating a New Film for Single-Use Bags: Growth Performance Studies with Animal and Human Cells. BioProcess Int. 14(3) 2016: 26–34; https://lne.e92.mwp.accessdomain.com/upstream-processing/upstream-single-use-technologies/evaluating-new-film-for-single-use-bags-growth-performance-studies-with-animal-and-human-cells.
48 Lawal AO, Ellis E. Differential Sensitivity and Responsiveness of Three Human Cell Lines HepG2, 1321N1 and HEK 293 to Cadmium. J. Toxicol. Sci. 35(4) 2010: 465–478; https://doi.org/10.2131/jts.35.465.
49 Kroll A, et al. Cytotoxicity Screening of 23 Engineered Nanomaterials Using a Test Matrix of Ten Cell Lines and Three Different Assays. Particle and Fibre Toxicol. 8, 2011: 9; https://doi.org/10.1186/1743-8977-8-9.
50 Park J-C, et al. Evaluation of the Cytotoxicity of Polyetherurethane (PU) Film Containing Zinc Diethyldithiocarbamate (ZDEC) on Various Cell Lines. Yonsei Med. J. 43(4) 2002: 518–526; https://doi.org/10.3349/ymj.2002.43.4.518.
Jennifer Kneitz is a technical-scientific employee, Maximilian Rotter is a technical-scientific employee, Gregor Dekevic is a PhD student, and Lukas Käßer is group leader at the Institute of Bioprocess Engineering and Pharmaceutical Technology (IBPT), Technische Hochschule Mittelhessen (THM) University of Applied Sciences, Giessen, Germany. Peter Czermak is managing director at IBPT and honorary professor at Justus-Liebig-University Giessen, Faculty of Biology and Chemistry, Giessen, Germany. Corresponding author Denise Salzig is group leader at IBPT; 49-641-309-2630; denise.salzig@lse.thm.de.