Flow-through anion-exchange (AEX) chromatography is used frequently in biopharmaceutical purification processes for reduction of net–negatively charged host-cell proteins (HCPs) and viruses as part of a validated viral clearance strategy (1, 2). AEX column chromatography is the technology most often used for electrostatic viral clearance, particularly in commercial-scale biopharmaceutical manufacturing, for which columns have a long-established history of reliable and well-understood performance (3). Still, validation of HCP and viral clearance by AEX columns in biopharmaceutical processes involves complexities that contribute significantly to operational and regulatory costs. Chromatography columns generally are used over tens to hundreds of purification cycles, with intervening cleaning procedures that can degrade resins. Biomanufacturers must be concerned about the potential for loss of HCP capacity or viral clearance due to fouling or degradation with reuse (4–7). An assessment of the effect of resin reuse on viral clearance thus is recommended generally by specific product (1, 5, 6).
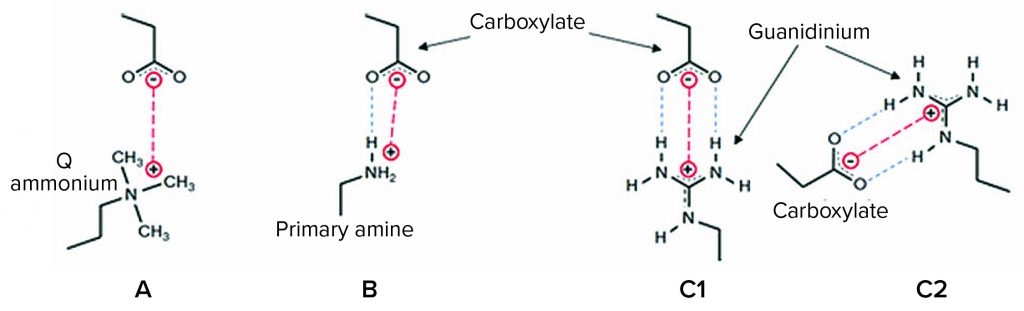
Figure 1: Interactions of anion-exchange (AEX) ligands with a carboxylate protein residue; (a) a quaternary ammonium (“Q”) ligand interacts electrostatically (red); (b) a primary amine ligand below its pKa (~10.6) interacts by a combination of an electrostatic interaction and a hydrogen bond (blue); (c1 and c2) guanidinium, the functional group of the natural cationic amino acid, arginine, forms either of two geometrically defined salt bridges (end-on and side-on, respectively) comprising an electrostatic interaction and two in-plane hydrogen-bonding interactions, forming a highly stable quasi-ring structure of six heavy atoms (19–22).
The introduction of single-use AEX technologies has illuminated the potential for reducing regulatory and operational costs associated with flow-through AEX chromatography (8–15). Physically resembling and operated like filters, single-use AEX products benefit from improved specific capacity and enhanced flow rates compared with columns because diffusive kinetics have been replaced by convective flow. Researchers note the potential for simplified operations, decreased processing times, and reduced buffer consumption, all of which improve economics relative to columns. Moreover, the single-use design obviates operational difficulties and validation and regulatory costs associated with cleaning over repeated use cycles.
Both AEX columns and AEX single-use products in wide use today suffer from important performance limitations across a range of bioprocess fluid conditions. Figure 1 illustrates interactions of some AEX ligands with a carboxylate protein residue — useful detail for understanding those limitations. For example, quaternary (“Q”) ammonium functional AEX resins and single-use media exhibit low capacity at elevated salt concentrations from electrostatic screening of the positively charged groups on the media, reducing the purely electrostatic interaction (Figure 1a). In purification processes with AEX polishing chromatography performed after cation-exchange (CEX) bind-and-elute chromatography, for example, that effect typically necessitates substantial product dilution to reduce the ionic strength of the CEX elution pool before the AEX step.
Primary amine functional AEX single-use products developed within the past decade are more salt tolerant (ST) because their primary amine ligands interact with anionic protein residues by hydrogen bonding in addition to electrostatic interaction (Figure 1b) (16). However, the primary amine ligand can begin to deprotonate in the range of pH between neutral and its pKa of ~10.6, reducing capacity (17), and it exhibits significantly impaired performance in multivalent buffers such as phosphate.
Although such trade-offs with respect to capacity and fluid conditions affect all commonly used AEX purification solutions, concerns are particularly acute with respect to single-use products. Whereas the excess cost of oversizing an AEX column to accommodate suboptimal fluid conditions can be borne over many use cycles, much of the value proposition from single-use solutions (in terms of reduced operational, validation, and regulatory costs) relies on replacing them for each batch. Thus, multiple investigators have expressed a need for single-use chromatography solutions to operate at high specific capacity over a broad range of conditions if they are to perform successfully as widespread replacements for production-scale columns (9, 18).
Here we examine HCP reduction and viral clearance by a new, hybrid ST AEX single-use product comprising two complementary AEX functional media: A Q-functional nonwoven and an ST guanidinium-functional membrane (Figure 2) (23). The Q-functional nonwoven reduces turbidity, DNA, and endotoxin and provides a portion of the product’s AEX capacity for HCP and virus reduction. The novel guanidinium functionality of the downstream AEX polishing membrane mimics arginine, one of the three positively charged naturally occurring standard amino acids that make up proteins. The guanidinium functional group of arginine has a resonance-delocalized positive charge in practically every biopurification setting because of its high pKa of 13.6 (24), and it has multiple coplanar pairs of hydrogen-bonding groups. It appears to have evolved in nature to provide a uniquely robust capability for interacting with the carboxylate residues of the two negatively charged amino acids — aspartic acid and glutamic acid — to form robust salt bridges based on two hydrogen-bonding interactions in addition to an electrostatic interaction (Figure 1c1 and 1c2). Indeed, the two geometrically defined guanidinium-carboxylate salt bridges are observed in nature to provide many of the intra- and interprotein interactions that stabilize the three-dimensional structures of individual proteins (19, 20) and protein–protein conjugates (21). The unique capability of guanidinium to bind carboxylate residues robustly appears to be reflected in our bioprocess data, through which remarkably consistent HCP reduction and viral clearance are observed across broad ranges of pH, ionic strength, and buffer type.
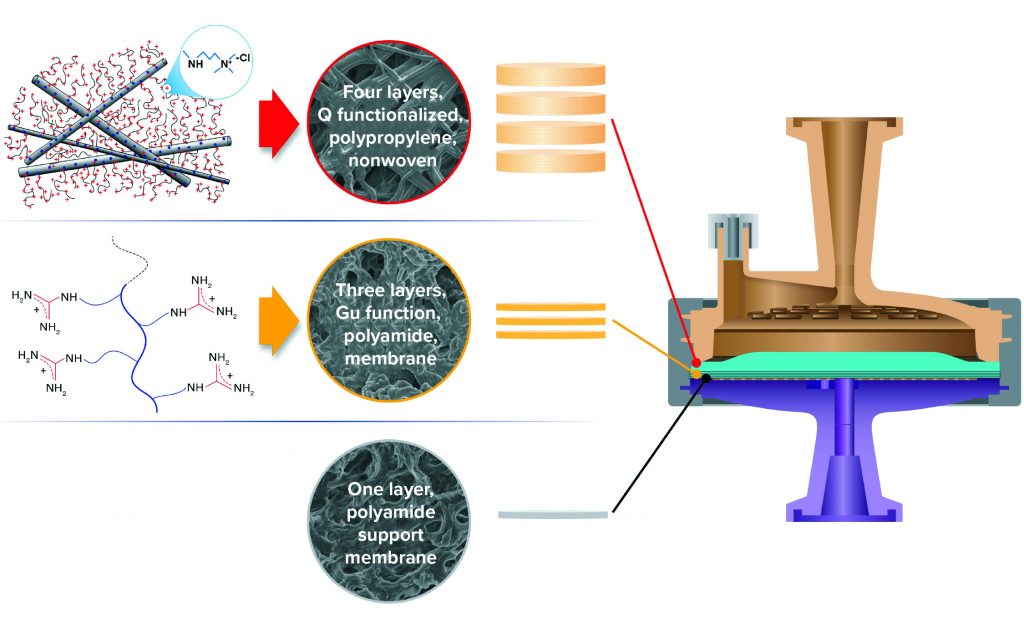
Figure 2: Hybrid media design of 3M Polisher ST single-use capsules, comprising a combination of four Q-functional nonwoven and three guanidinium-functional membrane AEX layers.
HCP Reduction, MAb Recovery: Comparisons with Q-Column
A monoclonal antibody (MAb)–containing Chinese hamster ovary (CHO) cell culture solution was gravity settled and sterile-filtered (0.2-µm 3M LifeASSURE PDA Series filter capsule), then loaded onto a Cytiva MabSelect SuRe protein A column. The protein A flow-through solution was collected, and the MAb was eluted from that column with 50 mM acetate buffer at pH 3.5. Quantification of HCP in the eluant fluid indicated an HCP concentration of <100 ppm with respect to the MAb. To increase the HCP load in the eluant, we prepared an HCP spiking solution by passing the protein A flow-through solution through a 3M Emphaze AEX Hybrid Purifier capsule to remove most of the DNA. Then we spiked the resulting HCP solution back into the MAb-containing eluant fluid to achieve a target HCP concentration of 500 ppm.
A series of simulated postcapture fluids in monovalent acetate-Tris buffers with pH ranging from 5.0 to 7.5 and conductivity ranging from 3.5 mS/cm to 20 mS/cm were prepared by adding 2 M Tris base and sodium chloride to aliquots of the spiked protein A eluant fluid. Simulated postcapture fluids in multivalent phosphate and citrate buffers were prepared by adding disodium phosphate or sodium citrate solutions and sodium chloride to aliquots of the spiked protein A eluant fluid. Table 1 details the pH, conductivity, and HCP concentrations of the challenge solutions.
3M Polisher ST BC1 capsules with an effective filtration area of 1 cm2 were challenged with each of the simulated postcapture solutions at a flow rate of 1 mL/min/cm2. HCP reduction and MAb recovery were measured at a MAb loading of 10 kg/m2. To obtain a performance comparison with a commercially available Q-column, we challenged a 5-mL Q-column (Cytiva HiScreen Capto Q column, column volume = 4.657 mL) with the postcapture solutions in a selection of the acetate-Tris buffers, prepared the same way as described above, at a flow rate of 0.43 column volumes (CV) per minute. HCP reduction and MAb recovery were measured at a MAb loading of 200 g/L. Before the challenge, each device type was preconditioned according to its manufacturer’s recommended procedure with a buffer matching the challenge solution buffer. Table 1 shows the number of replicates for each challenge.
Table 2 details mean values of HCP reduction and MAb recovery. For acetate-Tris fluids, Figure 3 provides contour plots of the HCP reduction values (shown in Table 2) as a function of pH and conductivity for the 3M Polisher ST capsule and the Q-column. With the exception of the challenge solutions in citrate buffer, the 3M Polisher ST capsule achieved greater than or equal to ~50% HCP reduction in all fluids, including multivalent phosphate buffer and other buffers with conductivity as high as 20 mS/cm.
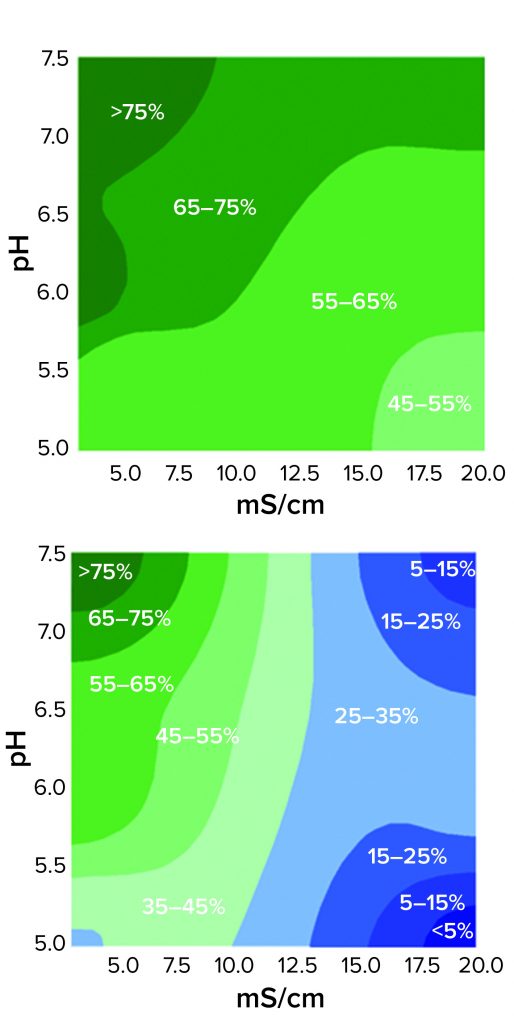
Figure 3: Contour plots of HCP reduction as a function of pH and conductivity for 3M Polisher ST capsules (top) and a Q-column (bottom) for acetate-Tris buffers with pH ranging from 5.0 to 7.5 and conductivity ranging from 3.5 to 20 mS/cm
The comparative robustness of HCP reduction by the guanidinium-functional single-use chromatography solution, relative to a conventional Q-column, is readily observable in Figure 3. Whereas ≥65% HCP reduction was achieved with the Q-column over only a narrow range at high pH and low conductivity, equivalent HCP reduction was achieved with the 3M Polisher ST capsule over a broad range of pH–conductivity combinations. MAb recoveries were >96% for all challenges and roughly equivalent between the Q-column and the single-use device.
The decreased HCP reduction by the 3M Polisher ST capsule in citrate buffer may not be surprising upon consideration of Figure 1 in combination with the chemical structure of citrate, a trivalent anion comprising three carboxylate groups. You would expect citrate to form the same salt bridges with guanidinium groups as the carboxylates of proteins and thus compete strongly with proteins for guanidinium binding sites. In practice, when the presence of citrate cannot be prevented, its competitive effect can be reduced by operating at higher overall conductivity and/or by preconditioning the media using a buffer that contains no citrate (see Regulatory Support File, 3M Polisher ST capsules, 3M Record-10-698362).
Viral Clearance: A Function of Buffer, pH, and Conductivity
3M Polisher ST BC1 capsules were challenged with four representative virus types, shown in Table 3, which were spiked into a series of monovalent and multivalent buffers having pH ranging from 5.0 to 7.5 and conductivity ranging from 3 to 20 mS/cm. The viral clearance testing was performed by an accredited laboratory. Before viral clearance testing, each capsule was sanitized with 1 M sodium hydroxide for 30 minutes and then preconditioned with the challenge buffer according to the manufacturer’s instructions. Each capsule was then loaded with 20 mL of virus-spiked challenge solution followed by 10 mL of chase buffer. The combined load and chase solutions were then submitted for viral quantitation. Two replicates were performed at each condition.
Table 4 summarizes the buffer conditions and measured viral clearance log-reduction values (LRVs) at each condition. Minute virus of mice (MVM), the most difficult of the viruses to clear, was tested at all conditions; the other viruses were tested at conditions selected to be the most challenging. In each case, the reported LRV is the minimum value of the two replicates. Viral clearance for all viruses was >5 LRV at high conductivity (20 mS/cm) and pH 5.5–7.5 in both monovalent and multivalent buffers.
The high viral clearance at 20 mS/cm is remarkable when compared with reported clearance at high conductivity for Q-columns (25). Viral clearance by the 3M Polisher ST capsules generally increased with increasing conductivity and pH. The isoelectric point of MVM is 5.2–6.2 (25). Thus, the lower viral clearance values for MVM at pH ≤ 5.5 are not surprising. Note that when compared with less ST AEX devices, this guanidinium-functional single-use device might enable the flexibility to achieve robust MVM clearance even at low pH (~5.5) by operating at high conductivity (≥10 mS/cm).
Reduction and Recovery in the Presence of Turbidity
When low-pH viral inactivation is used as a viral clearance step after protein A capture chromatography in MAb purification processes, significant fluid turbidity often is generated. That results from protein–protein and protein–DNA aggregate precipitation when pH adjustment of the fluid causes proteins to pass through their isoelectric points. Often, the fluid needs to be clarified before polishing chromatography steps.
To test the ability of the hybrid AEX media combination from Figure 2 to operate in a turbid environment, we prepared simulant turbid postcapture challenge fluids at pH 5.5 and 7.0 and with turbidities of ~40 and ~20 NTU. To prepare a protein A eluant stock fluid, a MAb-containing CHO cell culture solution was gravity-settled and sterile-filtered (0.2-µm 3M LifeASSURE PDA Series filter capsule), then loaded onto Cytiva MabSelect SuRe protein A column. The flow-through solution was collected, and the MAb eluted from the column with 50 mM acetate buffer at pH 3.5, resulting in a protein A eluant containing 22 mg/mL MAb. For preparing a ~20 NTU challenge solution, a typical procedure was to spike 10 mL of the protein A flow-through solution into 100 mL of protein A eluant and then adjust the pH of the spiked eluant to 7.0 using a 2 M Tris base. While turbidity was being measured, more flow-through solution was added until the turbidity reached ~20 NTU. For preparing a ~40-NTU challenge solution, a typical procedure was to spike 8 mL of the protein A flow-through solution into 100 mL of the protein A eluant and then adjust the pH of the spiked eluant to 5.5 with a 2 M Tris base. While turbidity was being measured, more of flow-through solution was added until turbidity reached ~40 NTU. Table 5 shows the final pH, conductivity, and turbidity, along with MAb, HCP, and DNA concentrations for each of the resulting challenge solutions.
In one set of trials, HCP and DNA reduction and MAb recovery were measured while monitoring pressure drop across the capsules. 3M Polisher ST BC1 capsules were preconditioned with buffer of the same pH and conductivity as a challenge fluid, according to manufacturer instructions, then challenged with two of the turbid challenge fluids at a flow rate of 1 mL/min/cm2 to a MAb loading of 10 kg/m2. Two replicates were performed with each challenge solution. Table 6 lists HCP and DNA reduction, MAb recovery, and initial and final pressure drops for the challenges. For all replicates with both turbid challenge solutions, HCP reduction was >50%, DNA reduction was >4 LRV, and MAb recovery was >97%. Moreover, the pressure drop across the capsules did not increase appreciably during the 10-kg/m2 load, indicating that the Q-functional nonwoven adequately managed the incoming turbidity.
In another trial, a preconditioned 3M Polisher ST capsule was turbidity challenged using the same flow rate and loading as above. Turbidities were measured for the protein A eluant solution before spiking and pH adjustment, the spiked and pH-adjusted challenge solution, and the filtrate. Table 7 lists the results of this challenge, and it is evident that filtration removed the excess turbidity generated by pH adjustment and spiking of the challenge fluid and restored a turbidity similar to that of the starting protein A eluant.
In a final set of trials, 3M Polisher ST BC1 capsules were challenged with samples of a turbid and highly contaminant-loaded postcapture-simulant fluid spiked with MVM or xenotropic murine leukemia virus (XMuLV). The viral clearance testing was performed by an accredited laboratory. Before viral clearance testing, each capsule was sanitized with 1 M sodium hydroxide and then preconditioned with acetate-Tris buffer at pH 5.5, according to the manufacturer’s instructions. Then each capsule was challenged at a flow rate of 1 mL/min/cm2 to a MAb loading of10 kg/m2 with the virus-spiked challenge solution, followed by 10 mL of chase buffer. Viral clearance testing was performed on two fractions of the filtrate for each capsule: the first 5 kg/m2 of MAb load and then the second such load including the chase buffer. Two replicates were performed for each virus type. Table 8 lists purification and viral clearance results, with each reported viral clearance value being the lower of the two replicates. Viral clearance for both viruses was >5 LRV at loadings up to 10 kg/m2.
Because the turbid challenge fluids were prepared by spiking a protein A eluant with protein A flow-through solution, they contained high levels of HCP and DNA contaminants, as quantified in Table 5. The robust reduction of HCP and DNA and viral clearance at 10 kg/m2 loading is thus notable. Additionally, the results suggest that this media combination might be used in turbid environments (e.g., following low-pH viral inactivation) without the need for pre-filtration.
Linear Scalability
3M Polisher ST capsules come as single-use capsules ranging in surface area from 1 cm2 to 1,020 cm2 and in target MAb loading capacity from 1 g to 1 kg, as detailed in Table 9. (Future commercialization is planned for 0.23‑m2 and 1.6-m2 capsules with target MAb loadings of 2.25 kg and 15 kg.) The linear scalability of the capsules with frontal media surface area was assessed by measurement of bovine serum albumin (BSA) protein dynamic binding capacity (DBC) as a simulant for HCP.
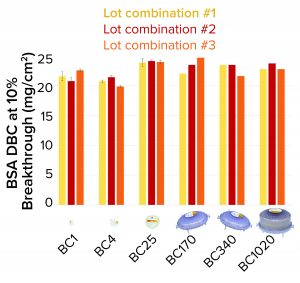
Figure 4: Bovine serum albumin (BSA) dynamic binding capacity (DBC) of 3M Polisher ST single-use capsules
3M Polisher ST capsules of each size were constructed with three different lot combinations of Q-functional nonwoven and guanidinium-functional membrane media. They then were challenged with 1-mg/mL BSA solutions in 25 mM Tris-HCl buffer at pH 8.0 containing 50 mM NaCl. The BSA capacity of each capsule was recorded as the cumulative mass of BSA bound at 10% breakthrough per unit of nominal media surface area. For BC1, BC4, and BC25 capsules, three replicates were performed for each media lot combination (a total of nine capsules of each size). For BC170, BC340, and BC1020 capsules, one replicate was performed for each media lot combination (a total of three capsules of each size). Figure 4 shows measured BSA DBC for all capsules and lot combinations. The BSA DBC per unit area is constant across all capsules, with a mean value of 22.6 mg/cm2 and a standard deviation of 1.4 mg/cm2.
To improve the accuracy of scaling, we calculated the relative scaling factors for the various capsules based on their measured BSA DBC. We used BC340 as the reference capsule and assigned a relative scaling capacity of 340 (equal to its nominal surface area in cm2). For each other capsule, the measured BSA DBC values across all three lot combinations were averaged, and the relative scaling factor was computed as
where RSFi is the relative scaling factor of capsule i, Ai is the nominal surface area of capsule i, and DBCi and DBC340 are the mean values of the measured BSA DBC for capsules i and BC340, respectively. The relative scaling factors of the capsules appear in the rightmost column of Table 8. Those values might be more accurate than nominal surface areas for the purposes of performing capsule scaling estimates. They could account for small deviations of the capsule sizes from unitary nominal surface area values and the effects of compression at the media edges within the capsules.
Robust Flexibility and Simplicity
By functionalizing a porous membrane with guanidinium moieties, the novel AEX media detailed above mimics the natural amino acid, arginine. Compared with commonly used Q-functional column resins, a hybrid single-use AEX device containing the guanidinium-functional membrane displays robust HCP reduction in a number of monovalent and multivalent buffers and across a broad range of pH and conductivities up to 20 mS/cm.
Viral clearance is similarly robust across a broad range of fluid conditions. The remarkable invariance of HCP and viral clearance with respect to fluid conditions is attributed to the unique, geometrically defined salt bridge formed between guanidinium and the anionic carboxylate residues of proteins.
By layering the guanidinium-functional membrane with an upstream Q-functional nonwoven, it is possible to construct AEX single-use devices that can operate in the turbid fluids often observed in MAb processes following low-pH viral inactivation. Note the ability of the hybrid device to achieve significant HCP and DNA reduction, even from highly contaminated fluids, at loadings of at least 10 kg/m2, which is consistent with estimates of the required capacity for single-use AEX solutions to compete favorably with multiuse columns at commercial scale (9).
Our results highlight the potential for substantial simplification of downstream polishing by replacing complex, buffer-hungry, condition-limiting AEX resin columns with simple, small-footprint, single-use chromatography devices. They offer a high degree of flexibility in buffer types and fluid conditions, providing HCP and viral clearance even in the presence of turbidity. Indeed, other investigators working with prototypes of the single-use AEX device have been able to simplify their MAb purification processes significantly in turbid postviral inactivation fluids by eliminating a unit process and avoiding the process fluid dilution required for an alternative commercial, non-ST AEX single-use device (26).
Acknowledgments
The authors thank Dr. Masa Nakamura and Blake Berg, who prepared all the CHO cell cultures used in the studies herein. Dr. Frederick LaPlant, Dr. Alexei Voloshin, Dr. Angelines Castro Forero, and Jacob Calhoun also are acknowledged for significant and valuable contributions to this work.
References
1 ICH Q5A(R1). Viral Safety Evaluation of Biotechnology Products Derived from Cell Lines of Human or Animal Origin. International Council for Harmonisation of Technical Requirements for Pharmaceuticals for Human Use: Geneva, Switzerland, 1999: https://database.ich.org/sites/default/files/Q5A%28R1%29%20Guideline_0.pdf.
2 CBER. Points to Consider in the Manufacture and Testing of Monoclonal Antibody Products for Human Use. US Food and Drug Administration: Rockville, MD, 1997; www.fda.gov/downloads/Biologics BloodVaccines/GuidanceCompliance RegulatoryInformation/recommendationsforManufacturers/ UCM153182.pdf.
3 Liu HF, et al. Recovery and Purification Process Development for Monoclonal Antibody Production. mAbs 2(5) 2010: 480–499; https://dx.doi.org/10.4161/mabs.2.5.12645.
4 Close EJ, et al. Fouling of an Anion Exchange Chromatography Operation in a Monoclonal Antibody Process: Visualization and Kinetic Studies. Biotechnol. Bioeng. 110(9) 2013: 2425–2435; https://doi.org/10.1002/bit.24898.
5 Norling L, et al. Impact of Multiple Re-Use of Anion-Exchange Chromatography Media on Virus Removal. J. Chrom. A 1069(1) 2005: 79–89; https://doi.org/10.1016/j.chroma.2004.09.072.
6 O’Leary RM, et al. Determining the Useful Life of Chromatography Resins. BioPharm Int. 14(9) 2001: 10–18.
7 Kelley BD, Jakubik J, Vicik S. Viral Clearance Studies on New and Used Chromatography Resins: Critical Review of a Large Dataset. Biologicals 36(2) 2008: 88–98; https://doi.org/10.1016/j.biologicals.2007.08.001.
8 Boi C. Membrane Adsorbers as Purification Tools for Monoclonal Antibody Purification. J. Chrom. B 848(1) 2007: 19–27; https://doi.org/10.1016/j.jchromb.2006.08.044.
9 Gottschalk U. Bioseparation in Antibody Manufacturing: The Good, the Bad, and the Ugly. Biotechnol. Prog. 24(3) 2008: 496–503; https://doi.org/10.1021/bp070452g.
10 Vicente T, et al. Anion-Exchange Membrane Chromatography for Purification of Rotovirus-Like Particles. J. Membrane Sci. 311(1–2) 2008: 270–283; https://doi.org/10.1016/j.memsci.2007.12.021.
11 Varadaraju H, et al. Process and Economic Evaluation for Monoclonal Antibody Purification Using a Membrane-Only Process. Biotechnol. Prog. 27(5) 2011: 1297–1305; https://doi.org/10.1002/btpr.639.
12 Orr V, et al. Recent Advances in Bioprocessing Application of Membrane Chromatography. Biotechnol. Adv. 31(4) 2013: 450–465; https://doi.org/10.1016/j.biotechadv.2013.01.007.
13 Weaver J, et al. Anion Exchange Membrane Adsorbers for Flow-Through Polishing Steps: Part I — Clearance of Minute Virus of Mice. Biotechnol. Bioeng. 110(2) 2013: 491–499; https://doi.org/10.1002/bit.24720.
14 Miesegaes GR, et al. Viral Clearance By Flow-Through Mode Ion Exchange Columns and Membrane Adsorbers. Biotechnol. Prog. 30(1) 2014: 124–131; https://doi.org/10.1002/btpr.1832.
15 Nascimento A, et al. Polishing of Monoclonal Antibodies Streams Through Convective Flow Devices. Separation and Purific. Tech. 132, 2014: 593–600; http://dx.doi.org/10.1016/j.seppur.2014.06.005.
16 Yonamine Y. Polymer Nanoparticle–Protein Interface: Evaluation of the Contribution of Positively Charged Functional Groups to Protein Affinity. ACS Appl. Mater. Interfaces 5(2) 2013: 374–379; https://doi.org/10.1021/am302404q.
17 Fischer-Fruholz S, Zhou D, Hirai M. Sartobind STIC® Salt-Tolerant Membrane Chromatography. Nat. Meth. 7(12–13) 2010: 950; https://doi.org/10.1038/nmeth.f.319.
18 Langer ES. Industry Adoption of Membrane Adsorbers: Fastest-Growing Little Segment in Bioprocessing. BioProcess Int. 11(10) 2013: 16–19; http://lne.e92.mwp.accessdomain.com/downstream-processing/filtration/industry-adoption-of-membrane-adsorbers-347331.
19 Singh J, et al. J. The Geometries of Interacting Arginine-Carboxyls in Proteins. FEBS Lett. 224(1) 1987: 161–171; https://doi.org/10.1016/0014-5793(87)80441-6.
20 Donald JE, Kulp DW, DeGrado WF. Salt Bridges: Geometrically Specific, Designable Interactions. Proteins 79(3) 2011: 898–915; https://doi.org/10.1002/prot.22927.
21 Yoshizawa T, et al. Structural Basis for Inhibition of Xyloglucan-Specific Endo-β-1,4-Glucanase (XEG) By XEG-Protein Inhibitor. J. Biol. Chem. 287(22) 2012: 18710–18716; https://dx.doi.org/10.1074%2Fjbc.M112.350520.
22 Rice A, Wereszczynski J. Probing the Disparate Effects of Arginine and Lysine Residues on Antimicrobial Peptide/Bilayer Association. Biochim. Biophys. Acta 1859(1) 2017: 1941–1950; https://doi.org/10.1016/j.bbamem.2017.06.002.
23 US Patent US 2018/0257042 A1. Filtration Medium Sequence for Biomaterial Purification. USPTO: Washington, DC, 13 September 2018; https://patentimages.storage.googleapis.com/01/22/f3/33b98357fc0ed8/US20180257042A1.pdf.
24 Vazdar M, et al. Arginine “Magic”: Guanidinium Like-Charge Ion Pairing from Aqueous Salts to Cell Penetrating Peptides. Acc. Chem. Res. 51(6) 2018: 1455–1464; https://doi.org/10.1021/acs.accounts.8b00098.
25 Strauss DM, et al. Understanding the Mechanism of Virus Removal By Q Sepharose Fast Flow Chromatography During the Purification of CHO-Cell Derived Biotherapeutics. Biotechnol. Bioeng. 104(2)2009: 371–380; https://doi.org/10.1002/bit.22416.
26 Singh N, et al. Development of Adsorptive Hybrid Filters to Enable Two-Step Purification of Biologics. mAbs 9(2) 2017: 350–364; https://doi.org/10.1080/19420862.2016.1267091.
Corresponding author Jonathan Hester, PhD, is a senior technical specialist in the Separation and Purification Sciences Division at 3M, 3M Center, 236-1C-14 | St. Paul, MN 55144-1000, 1-651-737-4078; jfhester@mmm.com. Jennifer Heitkamp is an application engineering specialist, Matthew Peters is a senior application engineer, Zona Jokondo is an advanced application engineer, and Jerald Rasmussen, PhD, is a staff scientist at 3M; www.3Mlifesciences.com; www.3M.com. 3M, LIFEASSURE, and EMPHAZE are trademarks of 3M Company.