The therapeutic benefits of monoclonal antibodies (MAbs) have been demonstrated in recent decades with uncontestable success as treatments for human disease. Despite MAbs’ key features such as specificity, selectivity, and safety, the format has limitations (1, 2). Bispecific antibodies may overcome number of difficulties (3).
Multiple formats of bispecific antibodies have been developed, although only the κλ-body is fully human and devoid of linkers or mutations. It requires no genetic modifications of heavy and light chains and results in bispecific antibodies with natural sequences (4). Different affinity chromatography steps have been developed for purification of bispecific MAbs.
However, development of a nonaffinity–based platform leads to more cost-effective production processes. The advent of hydrophobic cation-exchange resins, often referred to as mixed mode, provides opportunities to reduce the number of affinity steps in a platform process. Establishing protocols for such chromatography media required extensive screenings in the past. However, the advent of automated parallel chromatography, application of design of experiments, and use of prepacked columns accelerates this process.
Material and Methods
Bispecific Antibodies: A κλ-body was produced from recombinant Chinese hamster ovary (CHO) cells in a fed-batch fermentation process. The clarified fermentor content contained the three species — κκ monospecific MAb, λλ monospecific MAb, and κλ-body with a theoretical distribution of 25%, 25%, and 50%, respectively— and was partially purified by protein A chromatography. The pH of the protein A eluate was readjusted with 2.0 M Tris to pH 6.5, and the material was 0.22-µm filtered.
Analytical Chromatography: Analytical size-exclusion chromatography (SEC) was conducted using a TSKgel G3000SWxl (Tosoh Bioscience) 7.8-mm ID × 30-cm length column. 100 mM sodium phosphate buffer, pH 6.7, containing 100 mM sodium sulfate was used as liquid phase. The injected volume was 20 µL, and samples were injected without further preparation. The UV signal was traced at 280 nm.
Analytical cation-exchange chromatography (CEX) was conducted using a TSKgel SP-STAT (Tosoh Bioscience), 4.6-mm ID × 10-cm length column. The injected volume was 20 µL, and samples were injected without further preparation. The different monospecific and bispecific species were separated in a linear gradient from 5-mM sodium phosphate, pH 6.0, to 100 mM sodium phosphate and 500 mM sodium chloride, pH 6.0.
All columns were connected to analytical HPLC instruments. The applied flow rate was 1 mL/min. UV absorbance was recorded at 280 nm.
Preparative Chromatography: TOYOPEARL Butyl-600M and TOYOPEARL Phenyl-600M (Tosoh Bioscience) were used for preparative-scale hydrophobic interaction chromatography (HIC). 1 M ammonium sulfate in 100 mM sodium phosphate, pH 7.0, was used for column loading. The protein A elute pool was diluted 1:1 (v/v) with a 2× concentrated stock solution of the loading buffer. 10 mM sodium phosphate at pH 7.0 was used for elution.
TOYOPEARL MX-Trp-650M (Tosoh Bioscience) is a hydrophobic cation exchanger, herein simply referred to as a mixed-mode resin. 100 mM sodium acetate or sodium phosphate was chosen according to the applied pH. Product elution in linear gradient experiments was accomplished by addition of 500 mM sodium chloride to the binding buffer at constant pH. Buffers for step-elution experiments were substituted with sodium chloride to reach the required conductivity.
Dynamic binding capacities were determined with 6.6 mm ID × 2 cm/L Omnifit columns (Diba Industries, Cambridge, UK), which were packed with the different resins. The feed stream was diluted to 1 g/L protein and loaded at 150 cm/h. UV absorbance was monitored at 280 nm. The resulting breakthrough curves were used to calculate the dynamic binding capacity at 10% breakthrough.
Subsequent scouting experiments were conducted with 1 cm ID × 7.5 cm/L Omnifit columns. Alternatively, 8 mm ID × 10 cm L MiniChrom prepacked columns were used. A 50–column-volume linear gradient was applied with all resins and conditions. Buffers for preparative HIC and mixed mode were adapted from analytical chromatography. Collected fractions were analyzed with analytical CEX. Subsequent step-elution gradients were developed according to the scouting results. All resins were flushed with 200 mM sodium hydroxide after every cycle, followed by reequilibration.
Results and Discussion
Process Analytical Chromatography: Monospecific MAbs in the protein A elute pool eluted in a uniform peak in SEC. The retention of this peak corresponded to a molecular weight of 150 kDa. The presence of κ- or λ-light chains does not alter the average molecular weight of a MAb to an extent visible in SEC. The relative aggregate content of the protein A elute pool was below the target of 1%. Thus, optimization for further aggregate removal was not pursued.
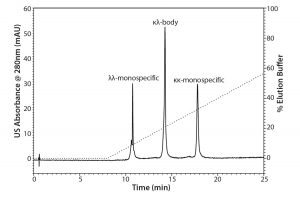
Figure 1: Analytical separations of the protein A elute pool containing the κκ-monospecific MAb, the κλ-body, and the λλ-monospecific MAb by CEX chromatography mode using TSKgel SP-STAT
Baseline separation of the three MAb variants was achieved with CEX chromatography using TSKgel SP-STAT (Figure 1). A comparison with the reference ratios indicated that the λλ-monospecific MAb eluted first, followed by the κλ-body and the κκ-monospecific MAb. Analytical CEX was used as the method of choice for process analysis during the scouting and evaluation of the preparative chromatography.
Scouting of Intermediate Downstream Processing: Because a one-step purification of bispecific MAbs using HIC has been described previously (5), HIC was the first chromatographic mode to be evaluated for separation of the κλ-body from the monospecific MAbs after an initial protein A capture step. Linear gradient scouting experiments were performed on TOYOPEARL Phenyl-600M and TOYOPEARL Butyl-600M for separation of κκ-monospecific MAb, λλ-monospecific MAb, and the κλ-body present in the protein A eluate pool. Peak elution from Phenyl-600M and Butyl-600M started when approximately 50% of the gradient was reached. Resolution using Butyl-600M was greater than using Phenyl-600M, with the κκ-monospecific MAb and the κλ-body resolved to baseline. Thus, Butyl-600M was selected for subsequent optimization experiments.
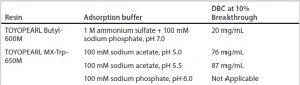
Table 1: Dynamic binding capacities (DBCs) of TOYOPEARL Butyl-600M and TOYOPEARL MX-Trp650M; the dynamic binding capacity of TOYOPEARL MX-Trp-650M in 100 mM sodium phosphate buffer, pH 6.0, cannot be determined through the applied breakthrough curve measurements because the λλ monospecific MAb does not adsorb to the resin.
By contrast with analytical CEX chromatography experiments, baseline resolution could not be achieved at process scale using CEX chromatography resins. A combination of HIC and IEC interactions may provide sufficient selectivity to accomplish separation of both monospecific MAbs and the κλ-body. A hydrophobic CEX (mixed mode) resin was therefore evaluated.
Chromatographic scouting runs of the separation of the κκ-monospecific MAb, the λλ-monospecific MAb, and the κλ-body on the mixed mode resin TOYOPEARL MX-Trp-650M have been performed in a linear sodium chloride gradient at pH 5.0, pH 5.5, and pH 6.0. At pH 5.0 and pH 5.5, all components of the protein A elute pool adsorbed to the resin, and protein eluted in the linear sodium chloride gradient in three separate peaks. At pH 6.0, the flow-through fraction contained λλ-monospecific MAb, whereas the κλ-body and the κκ-monospecific MAb were adsorbed to the resin (Figure 2). Table 1 shows the dynamic binding capacities of TOYOPEARL Butyl-600M and the mixed mode resin TOYOPEARL MX-Trp-650M.
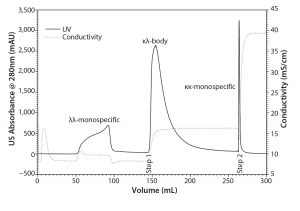
Figure 3: Separation of the protein A eluate pool on the mixed mode resin TOYOPEARL MX-Trp-650M in a step-gradient elution
Step-Gradient Elution Mixed-Mode and Hydrophobic Interaction Chromatography: Mixed-mode chromatography and HIC discriminated the κλ-body and the monospecific MAbs with orthogonal retention criteria. The selective flow-through of the λλ-monospecific MAb observed at pH 6.0 in the scouting experiments with TOYOPEARL MX-Trp-650M provided an opportunity to develop an efficient step-elution protocol for purification of the κλ-body. Figure 3 shows a chromatogram of a step-elution separation at pH 6.0. 50-mg protein/mL of the mixed-mode resin was loaded. Loaded amounts of bispecific and monospecific MAbs were well above load amounts reported for a strong CEX resin (6). The λλ-monospecific MAb flowed through the column without adsorbing to the mixed-mode resin. The κλ-body was recovered in the first sodium chloride step elution. The κκ-monospecific MAb was washed off during cleaning-in-place. The chromatography was performed at a linear flow rate of 300 cm/h, which allowed for fast processing. κλ-body purity was approximately 65%. The majority of the remaining contamination was κκ-monospecific MAb.
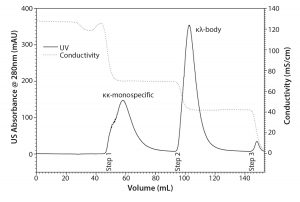
Figure 4: Purification of the κλ-body from the mixed mode step 1 eluate pool with TOYOPEARL Butyl-600M in a step-gradient approach; 99.5 % pure κλ-body is recovered during step 2.
Hence, a subsequent purification step was required. Selectivity of TOYOPEARL Butyl-600M is less susceptible to variations in conductivity and pH. Besides, the use of HIC adds another orthogonal separation criterion to the process. This is advantageous with regard to other process-related impurities, such as viruses and DNA. The TOYOPEARL MX-Trp-650M step 1 eluate pool containing κλ-body was loaded onto a TOYOPEARL Butyl-600M column. Figure 4 shows the chromatogram of a step gradient using 1 M ammonium sulfate. The κλ-body was recovered at a purity of 99.5%. Although the protein A elute pool did not contain significant aggregate levels, even after a low pH hold for virus inactivation, both TOYOPEARL MX-Trp-650M and TOYOPEARL Butyl-600M can be used for aggregate removal at conditions similar to those of the operating conditions applied here (7). Hence, it can be expected that the applied conditions would provide aggregate removal if a particular κλ-body candidate contained a higher level of aggregates. This is especially important with regard to platform applicability.
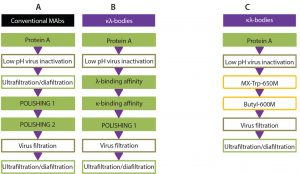
Figure 5: State-of-the-art downstream processing workflow for the purification of MAbs (A) and κλ bodies (B), compared with the new purification process for κλ bodies (C)
Comparable Purity, Lower Costs
Modern chromatography resins were evaluated for purification of a κλ-body. Hydrophobic CEX and HIC can replace a polishing step and two subsequent affinity-chromatography steps for purification of a κλ-body from monospecific MAb by-products (Figure 5). The three-step process using mixed mode and HIC chromatography showed comparable yields to a three-step affinity platform process currently used to purify a κλ-body. The excellent selectivity of TOYOPEARL MX-Trp-650M and TOYOPEARL Butyl-600M paves the way for future implementation at research, clinical, and commercial manufacturing scales. This approach combining reduced cost of goods and higher binding capacities offers an attractive new version of the purification process for the future manufacture of κλ-bodies.
References
1 Macor P, et al. Bispecific Antibodies Targeting Tumor-Associated Antigens and Neutralizing Complement Regulators Increase the Efficacy of Antibody-Based Immunotherapy in Mice. Leukemia 29(2) 2015: 406–414; doi:10.1038/leu.2014.185.
2 Dheilly E, et al. Selective Blockade of the Ubiquitous Checkpoint Receptor CD47 Is Enabled By Dual-Targeting Bispecific Antibodies. Mol. Ther. 25(2) 2017: 523–533; doi:10.1016/j.ymthe.2016.11.006.
3 Fischer N, Léger O. Bispecific Antibodies: Molecules That Enable Novel Therapeutic Strategies. Pathobiology 74(1) 2007: 3–14.
4 Brinkmann U, Kontermann RE. The Making of Bispecific Antibodies. MAbs 9(2) 2017: 182–212; doi: 10.1080/19420862.2016. 1268307.
5 Manzke O, et al. Single-Step Purification of Bispecific Monoclonal Antibodies for Immunotherapeutic Use By Hydrophobic Interaction Chromatography. J. Immunol. Methods 208(1) 1997: 65–73.
6 Hall T, et al. Alkaline Cation-Exchange Chromatography for the Reduction of Aggregate and a Mis-Formed Disulfide Variant in a Bispecific Antibody Purification Process. J. Chromatogr. B Anal. Technol. Biomed. Life Sci. 975, 2015: 1–8.
7 Vajda J, Mueller E, Bahret E. Dual Salt Mixtures in Mixed Mode Chromatography with an Immobilized Tryptophan Ligand Influence the Removal of Aggregated Monoclonal Antibodies. Biotechnol. J. 9(4) 2014: 555–565; https://doi.org/10.1002/biot.201300230.
Corresponding author Romain Dabre is product manager, process business at Tosoh Bioscience GmbH, Im Leuschnerpark 4, 64347 Griesheim, Germany; romain.dabre@tosoh.com. Judith Vajda was a senior laboratory specialist, and Egbert Müller is technical director at Tosoh Bioscience GmbH. Jean-Francois Depoisier was head of the downstream processing unit, Adrian Haines is head of process development sciences, and Alexandre Carron is senior bioprocess assistant, downstream processing unit, at Novimmune SA, Geneva, Switzerland.