Much has been written in recent years about the union of single-use systems (SUS) and process automation. These two technology initiatives have been prevalent within biopharmaceutical manufacturing over the past decade and are two of the most predominant advancements in biomanufacturing. A basic survey of industry media and conference topics corroborates that premise (1). However, efforts to combine them remain in the early stages of technological fulfillment, with much work to be done in realizing their synergistic benefits. Here I review the current state of this amalgamation of SUS and process automation, discuss possibilities moving forward, and look at the merits thereof.
Divergent Outputs
Each year, many conferences, publications, and events are devoted to SUS and process automation in biomanufacturing. Those focusing on SUS often are most easily recognizable because they feature either “Single- Use†or “Disposable†in their titles, but those focusing on process automation can be more inconspicuous.
One reason for the difference in apparent marketability is that the primary drivers for automation within the context of a manufacturing process are reduced levels of human interaction and/or intervention and increased levels of process control. The latter is often implied in more contemporary themes such as continuous quality verification (CQV), process analytical technology (PAT), process verification (PV), and statistical process control (SPC). Thus, the titles of associated conferences, publications, and events incorporating one or more of those themes may not at first glance reveal that they are related to process automation, but they are in fact very germane to the automation topic.
A Filtration Example: Ironically, SUS and automation historically have led to somewhat divergent outputs. Implementation of a common single-pass filtration system serves as an example. When typical multiuse systems (MUS) — those that comprise stainless steel housings and piping, perhaps in the form of a skid — are used for this process step, they often incorporate a fair degree of automation. Although inherently simple, such automation typically involves a means of flow control that is predicated on pressure feedback with flow paths directed by automated valves. Relevant process data are captured and logged in real time for each campaign, allowing for monitoring of process variability.
Most common SUS that accomplish the same basic process functionality comprise encapsulated filters, polymeric tubing, manually engaged connections to downstream process steps, and perhaps a biocontainer to capture effluent from filter flushing. In these systems, however, it is uncommon to find a pressure feedback loop. Flow typically is directed with manual pinch clamps, which offer limited provision to capture process data. So although single-use implementation may reduce process and product risks while enhancing operational flexibility in commonly understood ways, it takes a measured step backward in terms of process automation.
That recognition is not intended to diminish SUS in terms of their many well-accepted benefits. I simply point out that SUS implementation can result in a lesser degree of process automation than comes with a typical MUS used to accomplish the same operational step. Although many readers are familiar with the inherent benefits of both SUS and process automation technologies, briefly revisiting those below will set the stage for further discussion.
Drivers for Single-Use Technology
Reduction in Cost: The two fundamental ways to minimize manufacturing costs are by reducing initial capital investment and lowering operational costs. The former takes into account reduced equipment costs and facility requirements — cleanroom infrastructure, clean-in-place (CIP) skids, steam-in-place (SIP) utilities, and so on — as well as the smaller overall operational footprint that can facilitate operational aspects of multiproduct facilities.
Lowering operational costs comes from reduction or elimination of CIP and SIP processes as well as shortened set-up and campaign change-over times. Such costs are variable by nature. Although certainly in some instances, for example, the cost of a single-use consumable at a given operational scale may skew a cost analysis, many facilities for manufacturing phase 1–3 products and even commercial production may achieve a manufacturing cost benefit from SUS rather than MUS implementation. Not surprising, reduction of overall manufacturing cost remains a major driver for SUS implementation (1, 2).
Increased Speed of Implementation: Manufacturing operations based on SUS typically are quicker to implement than those based on MUS. Future modifications also are more rapidly achievable — e.g., those that may become necessary to keep pace with process improvements or scale adjustments. The main challenge to speeding up SUS implementation is often regulatory in nature (e.g., analyzing leachable and extractable substances) (1, 2). However, the industry is taking steps to streamline efforts in that regard.
Meanwhile, some regulatory-related endeavors required of biomanufacturers using MUS will be mitigated to a degree with SUS. An excellent example is sterilization validation. SUS suppliers must be able to substantiate the sterility of their systems when appropriate, thereby effectively removing that task from the list of things that end users must accomplish (other than through a supplier technical diligence visit and thorough, documented quality audit). This further improves the speed of implementation.
Reduction in Risk: When properly conceived, designed, and implemented, SUS can mitigate the associated process and product risk for a given biomanufacturing process. Some primary considerations in this regard are increased levels of sterility assurance, elimination of cross-contamination concerns, and an ability to use closed systems. For some analogous MUS-based operations the latter is impossible.
Drivers for Process Automation Technology
Reduction in Risk: Automation can effectively provide a level of continuous quality assurance through, for example, real-time monitoring of key process and product quality attributes. Further, decreased human interaction and/or intervention with a given manufacturing process mitigates the occurrence of human error.
Increased Level of Process Control: In addition to other attributes covered above, process automation typically provides heightened levels of process repeatability. Further, process control is by nature inversely proportional to risk (3); process and product risks are reduced with increased process control.
Agglomerated Drivers
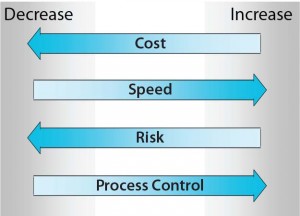
Figure 1: The four agglomerated drivers for
single-use systems (SUS) and process
automation technologies that can be
considered most crucial in evaluation and/or
implementation for most process applications
— reduction in cost (Cost), increased speed of
implementation (Speed), reduced level of risk
(Risk), and increased level of process control
(Process Control) — are depicted here as
directional arrows toward decrease or increase.
Reduction in risk is common to both SUS and process automation technologies. Combining the drivers of both technologies can lower costs, increase speed of implementation, reduce risk, and improve process control (Figure 1). These technologies perhaps share other ancillary benefits; however, those four (cost, speed, risk, and process control) can be considered the most crucial agglomerated drivers relevant to evaluation and/or implementation for most real-world manufacturing process applications.
A Mixing Example: As an in-depth, example of the possible divergent outputs of SUS and process automation technology initiatives have led to in historically prevalent implementations, consider mixing applications. Typical MUS mixing systems incorporate a fair amount of process control with programmable logic controllers, and are often recipe driven from start to finish. It is fair to note that some of that process control is provided for steps that are simply not required for SUS (e.g., SIP); however, the actual mixing process itself also typically is recipe driven. Furthermore, electronic batch data are collected and recorded throughout each operation.
With the advent of single-use mixing (and still common today in many systems), process control was reduced to a simple rheostat and timer with a knob and a switch. Recent single-use mixing systems provide more advanced functionality — which is typically offered, however, through stand-alone components that are not otherwise incorporated into an overall process control system.
Applying the four agglomerated drivers from Figure 1 to selection of a common single-use mixing system — as opposed to a common MUS for accomplishing the same goal — yields the following analysis.
Cost: For this analysis, assume moderate process volumes of 200– 500 L, a moderate run rate of two batches per week, and a relatively simple process. Under those assumptions, the cost analysis typically comes out in favor of SUS. It should be noted, however, that consumables associated with this single-use operation can be costly given its complexity. That can lessen the relative cost benefits associated with selection of a single-use mixing solution.
Speed: Rapid implementation is generally a notable advantage associated with SUS in mixing applications. When biomanufacturers work with responsive suppliers, it should be more expedient for them to implement a single-use mixing solution than a comparable MUS. Speed of implementation not only accounts for delivery, but also installation (e.g., utility hookups) as well as commissioning and validation activities.
Risk: Both multiuse and single-use mixing implementations, presented here as typical manifestations thereof, can present unique operational process and product risk factors while accomplishing largely the same functionality. The two systems’ different operational characteristics ultimately drive those differing process and product risk factors. Risk certainly warrants a more in-depth analysis than what is covered here, but I offer a brief exploration below.
The unique operational process and product risk factors most commonly associated with MUS are sterilization, and cross contamination, both of which are complicated by systems that contain challenging geometry and relatively high levels of design complexity. In single-use mixing applications, the typical unique process and product risk factors to be considered include deployment and system integrity. The former describes alignment and installation of an impellor/agitation device as well as the associated biocontainer and fluid paths. Manual processes can be somewhat complex. Simple systems can be handled relatively easily by a well-trained operator, but the level of process risk increases with larger, more complex, or ill-conceived systems. System integrity is most often challenged by the litany of methods used to transfer motion into a closed single-use process envelope: e.g., use of friction bearings or introduction of a reinforced shaft into part of the system. Nearly all such technologies warrant unique risk considerations.
With all those unique risk factors considered for this application of a relatively simple mixing system, the single-use option typically represents a lower risk profile.
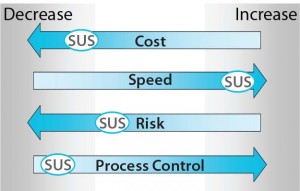
Figure 2: Outputs of an analysis assuming
selection of a common SUS mixing
implementation relative to a comparable
multiuse system (MUS) implementation
assessed against the four agglomerated drivers
derived from Figure 1
Process Control: Of the two systems considered here, the single-use mixer represents a lower level of process control. Figure 2 depicts the outputs of the above analysis for four agglomerated drivers (Figure 1) assuming selection of a common single-use mixing system. Note that each marker (an oval designated “SUSâ€) is represented relative to the continuum presented within the figure.
Cost is represented as a decrease on the continuum when comparing SUS and MUS implementation for this application. The decrease achieved with the single-use mixer may not be as profound as initially expected, however, because of the expense of associated consumables, which as previously noted can be high.
The relative speed of implementation is represented as a marked increase that comes with selecting a single-use rather than multiuse system for this mixing application. And risk is represented as decreasing with SUS selection from that with MUS implementation for this application. The position on the continuum suggests only a slight decrease in process and product risk, with the magnitude thereof being mitigated by the unique factors outlined above.
In selecting a common single-use mixing implementation, the pronounced departure from achieving success for the drivers in Figure 2 lies in process control. The single-use mixer considered represents a markedly lower level of process control than a typical multiuse mixer, which is a divergent output against the established, agglomerated drivers.
Of course, that analysis can be skewed by making adjustments to the SUS and MUS implementations considered. Often, however, those adjustments can have unintended consequences. For example, increasing the level of process control afforded by the single-use mixer would tend to erode both its cost advantage (typical single-use sensors are expensive) and compromise the risk analysis (because increased system complexity usually leads to increased process risk).
Hybrid Systems: A New Definition
In relation to SUS or MUS in biopharmaceutical manufacturing operations, the term hybrid system usually refers to a process implementation using SUS for certain portions and MUS for others. Common examples include the use of SUS to store media before feeding a multiuse bioreactor and/or the use of SUS to prepare buffers for multiuse chromatography columns.
In broad terms, a new definition of a hybrid system would be one in which smart, integrated infrastructure is incorporated as a multiuse system interfaces directly with renewable and simple fluid-contact surfaces incorporated as a single-use system. This is no revolutionary direction for the industry; steps have been taken progressively in this direction, and in some other discreet processes (e.g., filling applications) such an approach is becoming fairly standard. However, it makes sense to explore this new definition of hybrid systems and how it can relate to the next generation of single-use–enabled operations for biomanufacturing. In short, the new definition provides a number of advantages and can lead to an alignment of above-discussed divergent outputs from the SUS and process automation technology initiatives.
By limiting the scope of consumable components in a hybrid system through use of simple (“dumbâ€) single-use fluid-contact surfaces, a biomanufacturer can moderate the complexity, material diversity, and sheer amount of a given processing system that will be disposed of with each campaign. Doing so would have several positive effects:
- the cost of each consumable generally correlates to its scope and complexity, so limiting the number of consumable components helps to mitigate costs
- the environmental impact will be reduced when less material is discarded
- the process and product risks associated with SUS use are proportional to system complexity and material diversity, so a reduction in their scope also serves to mitigate those risks.
All three of those items are commonly cited as primary concerns among SUS end users (1, 2).
By a “dumb†single-use fluid contact surface, I mean one that largely serves only to provide a closed system. Functionality related to flow control, sensing functionality, and overall process control is accomplished by the integrated MUS infrastructure to the extent possible. Technological advances have been made that facilitate conception of such simple fluid-contact surfaces, although work remains to be done in this regard.
Examples of those advancements include pressure and flow sensors that do not contact fluid, both of which are currently available. Such noninvasive sensors allow for
- management of process-critical instruments within standard asset-tracking routines — rather than having to maintain a calibration record for each individual single-use sensor
- off-line calibration or sensor replacement in the case of an unexpected technical issue — rather than wholesale abandonment of a single-use consumable, or worse, the associated batch
- reduction in the number of fluid-contact materials, the proliferation of which can exacerbate efforts in relation to leachable/extractable substances.
Other items that can be included within an integrated MUS infrastructure include load cells and automated pinch and/or metering valves. They serve to enhance the automation possibilities of a hybrid system while simplifying associated consumables by, for example, omitting manual tubing clamps.
Additional benefits of this newly defined hybrid system include simplified and better controlled deployment of each simple, single-use, fluid-contact surface component. With a single-use system now simplified and the integrated MUS providing a defined fluid-path placement — through automated pinch clamps, for example — the resulting overall system will tend to be more repeatable and robust than it would have been otherwise. This approach addresses a unique process risk factor typically associated with SUS. Furthermore, simplifying SUS broadens the ability of end users to source items from multiple vendors. That will remain a challenge for more complex systems associated with certain unit processing operations such as bioreactors. But the resulting MUS infrastructure can effectively allow for open architecture related to many consumables associated with basic operations. And that mitigates yet another frequent user concern regarding sole sourcing (1).
An updated definition of hybrid systems seems to best facilitate integration of SUS and process automation. It should allow for next-generation systems that harness the operational advantages provided by each technology and deliver achievement in relation to all four drivers described above.
Smart Single-Use Process Solutions
To further the discussion of new hybrid systems, consider how they may manifest in mixing applications. A single-use mixing system that embraces the newly defined hybrid methodology (a “smart single-use process solutionâ€) would provide enhanced levels of process control without raising costs or risks associated with a given consumable, which such endeavors have historically done. That is accomplished with a smart integrated MUS infrastructure that includes a high degree of automation, advanced process control, and active process monitoring with data-acquisition capabilities, all accomplished through a single integrated human–machine interface (HMI). The scope of the associated SUS consumable(s) would remain limited through the use of non– fluid-contacting sensors included within the multiuse system. SUS deployment can be largely system controlled, further mitigating process risk typically associated with single-use mixing applications. And finally, the means by which motion is transferred to the closed single-use process envelope can be addressed to further reduce the consumable’s scope while addressing yet more unique process and product risk factors associated with single-use mixing applications.
Revisiting the mixing example — but assuming a technology platform that embraces the new hybrid-system definition — my assessment changes as follows.
Cost: The scope and complexity of the single-use consumable(s) have not changed despite the increased system capability obtained by incorporating smart integrated MUS infrastructure. Although the fixed MUS costs are likely to increase slightly, the recurring single-use costs will be remain unchanged from the initial assessment.
Speed: The speed at which this system can be brought online remains the same as well. Beyond simply evaluating the pace of implementation, note that this new hybrid system improves a company’s ability to rapidly change over between manufacturing campaigns. SUS deployment has been simplified and automated to some degree while administration of calibratable assets has been minimized. Even so, the speed is represented as nearly unchanged for the scope of this analysis.
Risk: A quantifiable shift from the initial analysis begins to be apparent in relation to risk. With an increased level of process control through automation, process and product risk have been decreased. Automating SUS deployment and addressing the manner in which motion is imparted to the closed system effectively mitigates two of the unique process and product risk factors associated with single-use mixing.
Process Control: As indicated above, the level of process control has dramatically improved by integrating automation and single-use technology.
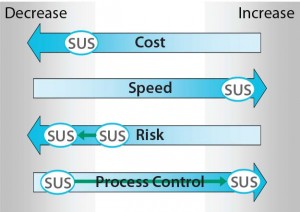
Figure 3: Effect of smart single-use process
solutions and the new definition of hybrid
systems on single-use mixing implementations
as assessed against the four agglomerated
drivers derived from Figure 1; shifts relative to
the original assessment in Figure 2 are
delineated by green arrows.
Figure 3 depicts outputs of a single-use mixing solution that embraces the newly defined hybrid system methodology. Green arrows represent shifts that have occurred from the assessment of a common single-use mixing solution in Figure 2. The two quantifiable shifts based on consideration of a smart single-use process solution lie in reduction of process and product risk and marked improvement of process control provided by the system overall. Both gains have been achieved without compromising the speed and cost benefits associated with the previously considered single-use mixing implementation. This shows how a system that embraces the new definition of hybrid by integrating SUS and process-automation technologies can facilitate achievement against the established agglomerated drivers and lead to alignment of the divergent outputs discussed above.
Moving Forward
Some of my statements are forward-looking, but they do represent the direction that is being taken by innovative SUS manufacturers and end users alike. The primary technical challenge lies in deploying advanced technologies both for single-use fluid contact components and those that serve to advance integrated MUS. The goal is to find an appropriate balance between consumable components and an integrated infrastructure, thereby enabling the next generation of smart single-use process solutions.
Development and manufacturing of next-generation integrated hybrid systems should be fueled by a desire to reap the benefits of converging SUS and process automation. This will serve to enhance many facets of biopharmaceutical production, which is a goal shared by all stakeholders alike.
The intent of this discussion is not to suggest that pervasive issues should limit SUS adoption in typical current manifestations. SUS have been one of the most prevalent technology initiatives within the biomanufacturing industry over the past 10 years for a good reason. They make a great deal of sense. However, as their development continues, we can expect to harness additional SUS benefits as they further align with process automation.
References
1 Caine B. BPI’s 2014 Single-Use End User Survey: How Do You Manage and Evaluate Single-Use Performance? BPSA 2014 Annual Meeting, SOCMA: Washington, DC.
2 Langer E. Bio-Plan’s 11th Annual Report on Market Trends in the Single-Use Industry. BPSA 2014 Annual Meeting, SOCMA: Washington, DC.
3 CDER/CVM/ORA. Guidance for Industry: PAT — A Framework for Innovative Pharmaceutical Development, Manufacturing, and Quality Assurance. US Food and Drug Administration: Rockville, MD, September 2004; www.fda.gov/downloads/Drugs/ Guidances/ucm070305.pdf. •
Max Blomberg is director of operations at Meissner Filtration Products, 1001 Flynn Road, Camarillo, CA 93012; 1-805-388-9911, ext. 552; max.blomberg@meissner