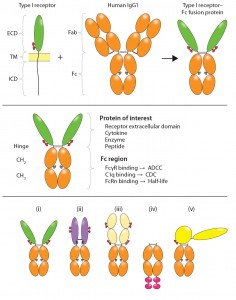
Figure 4: Structural variety of FDA-approved Fc-fusion proteins is illustrated. Typical receptor ECD- containing Fc fusions exhibit single-ligand specificity in bivalent fusion constructs such as (i) alefacept, and (ii) abatacept and belatacept (black dots in denote Cys–Ser mutations in the hinge); “cytokine traps” (iii) such as rilonacept, aflibercept, and ziv-aflibercept; and “peptibodies” (iv) such as the aglycosylated romiplostim with single-ligand specificity in multivalent fusion constructs (in which peptide mimetics represented by large red ovals are attached in tandem and separated by flexible spacers at the C-terminal end of IgG Fc); etanercept (i) and clotting factor Fc (v) FIX Fc and FVIII Fc, with single-ligand specificity in a monovalent fusion construct. Shown in yellow are domains of truncated FIX. Horizontal lines indicate interchain disulfide bonds. Glycosylation sites are indicated in the ligand-binding domains (small red ovals) and Fc domain (small gray ovals). This figure is reproduced and adapted with permission from (3).*
The potential therapeutic value of many proteins — including enzymes, receptors, cytokines, blood factors and peptides — can be realized by fusing them to the Fc region of human immunoglobulin G. Of the 46 monoclonal antibody (MAb) and MAb-derivative products approved by the FDA to date as human therapeutics, 10 are Fc-fusion proteins (Table 2). Among approved products, several structural variations are represented (Figure 4).
In BPI’s October 2014 issue, Part 1 of this review examined the structure and manufacturing of Fc-fusion proteins. It referred to 45 MAb and MAb-derivative products approved by the FDA. One additional Fc-fusion protein (Eloctate) was approved while this manuscript was in review. This month, we conclude with a detailed examination of such products that have received market authorization over the past 16 years. Tables, figures, and references are numbered continuously from Part 1.
Fc-Fusion Proteins As Drugs
Currently nine Fc-fusion proteins are FDA approved and marketed in the United States as biopharmaceutical products (Table 2). Other compounds are in late-stage clinical development (Table 3). For several clinical indications (e.g., autoimmune conditions such as rheumatoid arthritis), Fc-fusion proteins directly compete with MAbs that are designed to bind to similar molecular targets. Nine of the 10 approved Fc-fusion proteins are homodimers. They can be categorized into four groups based on ligand specificity (binding to one or multiple epitopes on a ligand molecule) and valency (stoichiometry of binding to ligand molecules):
- bivalent with single-ligand specificity
- monovalent with multiligand specificity
- multivalent with single-ligand specificity
- monovalent with single-ligand specificity.
Abbreviations Used Herein
ADCC: antibody-dependent, cell- mediated cytotoxicity | IL-1: interleukin-1 |
Ang: angiopoietin | Kd: equilibrium dissociation constant |
APC: antigen presenting cell | LFA-3: lymphocyte function-associated antigen 3 |
BAFF: B-cell activating factor | MAb: monoclonal antibody |
CDC: complement-dependent cytotoxicity | PEG: polyethylene glycol |
CHO: Chinese hamster ovary cells | PlGF: placental growth factor |
ECD: extracellular domain | RA: rheumatoid arthritis |
Fab: antigen binding, comprising VL, VH, CL, and CH1 domains | SLE: systemic lupus erythematosus |
Fc: effector, comprising hinge, CH2, and CH3 domains | TM: transmembrane domain |
FcRn: neonatal Fc receptor | TNF: tumor necrosis factor |
GLP-1: glucagon-like peptide-1 | TPO: thrombopoietin |
HEK-293: human embryonic kidney 293 cells | VEGF: vascular endothelial growth factor |
ICD: intracellular domain | VLDL: very low-density lipoprotein |
Group 1 — Single-Ligand Specificity in Bivalent Fusion Constructs:
Alefacept, etanercept, abatacept, and belatacept are examples of homodimeric molecules comprising single-ligand binding domains derived from receptor ECDs. The fusion molecules contain two ligand-binding sites and are thus functionally bivalent. They act as receptor antagonists. Alefacept (Astellas and Biogen Idec’s Amevive product) is the first biologic approved to treat patients with moderate-to-severe plaque psoriasis. It is composed of the first ECD of lymphocyte function- associated antigen 3 (LFA-3, CD58) fused to the human IgG1 Fc domain. Expressed on many cell types including antigen-presenting cells (APCs), LFA-3 is the primary coreceptor for CD2 on T cells and natural killer (NK) cells. The majority of T cells in psoriatic lesions are CD45RO+ memory effector cells that express a high level of cell-surface CD2. By binding to CD2 on those T cells, alefacept effectively prevents APC cross-talk with T cells and therefore inhibits T-cell activation (23). Alefacept has a circulating half- life of 12 days in humans (24).
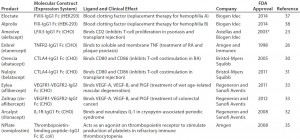
Table 2: FDA-approved therapeutic Fc-fusion proteins (* indicates a product withdrawn from the market in 2011)
Abatacept and belatacept (Bristol- Myers Squibb’s Orencia and Nulojix products, respectively) consist of the ECD of human cytotoxic T lymphocyte associated molecule-4 (CTLA-4) fused to the Fc domain. They were designed as receptor antagonists to block the interactions between CD80 or CD86 on APCs and CD28 on T cells, which provide the secondary costimulatory signal needed for T-cell activation. Abatacept incorporates the disulfide-linked homodimeric structure of CTLA-4, enabling the fusion protein to bind to CD86 with a stoichiometry of 1:2 (25).
That drug was developed by Bristol- Myers-Squibb and approved for the treatment of rheumatoid arthritis (26), with several trials ongoing for other indications. The company later developed a more potent, engineered version of CTLA-4 Fc (belatacept) for renal and liver transplant rejection (27). The new molecule contains two amino- acid substitutions (L104E and A29Y) in its CTLA-4 region, giving it higher affinity for both CD86 and CD80, and it is 10-fold more potent as measured by in vitro T-cell activation assays. Belatacept was approved in 2011.
Group 2 — Traps (Multiligand Specificity in Monovalent Fusion Constructs): Rilonacept, aflibercept, and ziv-aflibercept are examples of cytokine traps: homodimeric molecules made up of multiligand-binding domains derived from different receptor chains. Their ligand-binding domains combine functionally to form a single binding site, thus “trapping” a single ligand molecule between the two chains. The drugs are thus functionally monovalent and act as receptor antagonists.
Rilonacept (Sanofi Aventis and Regeneron’s Arcalyst product) is an example of a cytokine trap. Developed by Regeneron, it was approved in 2008 to treat cryopin-associated periodic syndromes (CAPS), a rare autoinflammatory disease. Using domains from different interleukin 1 (IL1) binding proteins, Regeneron fused the C-terminus of the IL1 receptor accessory protein (IL1RAcP) ligand-binding region to the N-terminus of the IL1R1 ECD, then fused this hybrid IL1 binding domain to human IgG1 Fc. Rilonacept is an IL1 antagonist with high affinity (Kd = 1.5 pM) and potency (IC50 = 6.5 pM). Its 8.6-day half-life enables weekly administration. By contrast, anakinra (Amgen’s Kineret product) is a recombinant non-glycosylated version of IL1RA produced in Escherichia coli that must be administered daily because of its rapid clearance in vivo (28).
Aflibercept (Sanofi Aventis and Regeneron’s Eylea product) is an antiangiogenic fusion protein designed to bind vascular endothelial growth factor A (VEGF-A), VEGF-B, and PlGF, all of which are implicated in tumor angiogenesis. The design of this molecule illustrates the use of protein engineering to combine domains of different receptors to overcome issues that were initially encountered with one or the other (29). Originally the VEGF trap molecule was created by fusing the first three domains of the VEGF receptor 1 to human IgG1 Fc. The protein was very potent when measured by in vitro tumor growth inhibition assays, but it exhibited a poor pharmacokinetic profile and nonspecific interactions that made it unsuitable as a clinical candidate. The developers deleted the entire high pI domain 1, thought to be responsible for nonspecific binding, and replaced the high pI domain 1, with one characterized by a lower pI and better binding (domain 3 from VEGF receptor 2), resulting in a final construct that actually improved the binding affinity in addition to lowering the pI (29).
Aflibercept thus has much improved pharmacokinetic properties and better antitumor activities, making it better than the sum of its parts. Developed by Regeneron, it was approved in 2011 (Eylea, formulated for intravitreal injection) to treat wet age-related macular degeneration. In 2012 the Zaltrap version (ziv-aflibercept, formulated for intravenous infusion) was approved for metastatic colorectal cancer. Net Eylea sales in the United States for 2012, its first full year on the market, were $800 million (3).
Group 3 — Peptibodies (Single- Ligand Specificity in Multivalent Fusion Constructs): Romiplostim (Amgen’s NPlate product) is a peptibody: a homodimeric peptide molecule that is specific for a single ligand. First identified using phage display technology, this 14–amino-acid peptide binds to thrombopoietin receptor (also known as c-Mpl on CD1110) (30), mimicking the activating effect of thrombopoietin (TPO). For the fusion construct, multiple copies of the ligand- binding peptide are fused in series to each C terminus of a human IgG1 Fc dimer. The first c-Mpl-binding peptide is separated from Fc by a five–amino- acid flexible glycine linker. It is followed by another, longer glycine linker before the second copy of the c-Mpl-binding peptide, making it functionally bivalent.
Binding of romiplostim to c-Mpl activates several signaling pathways, including tyrosine phosphorylation of c-Mpl and of proteins in the original transduction pathways JAK, STAT, and MAPK. By design, the active peptide shares no amino acid sequence homology to endogenous TPO, eliminating all risk of eliciting an immune response that cross- neutralizes endogenous TPO. Glycosylation is not required for activity of the peptide-binding moiety, nor is it required for the Fc to bind to FcRn (10). Consequently, romiplostim is produced in E. coli. The fusion molecule acts as a receptor agonist and is functionally bivalent. Developed by Amgen, romiplostim was approved in 2008 for treating thrombocytopenia in patients with chronic idiopathic thrombocytopenic purpura (31).
Group 4 — Etanercept and Clotting Factor Fc (Single-Ligand Specificity in Monovalent Fusion Construct): Etanercept (Amgen’s Enbrel product) is composed of the 75-kDa soluble ECD of the TNFα-receptor II (TNFR II) fused to the Fc domain of human IgG1. Developed by Immunex and approved by the FDA in 1998, the drug was acquired by Amgen in 2002. It was the first successful commercial example of a soluble receptor–Fc-fusion protein used as a therapeutic. The drug neutralizes both membrane-bound and soluble forms of TNFα, thus reducing the inflammatory downstream effects of TNFα. Dimeric etanercept binds to trimeric TNFα with a stoichiometry of 1:1 (32). The TNFR II receptor ECD contains both N- and O-linked oligosaccharides.
Etanercept was initially approved for treatment of rheumatoid arthritis (RA); in addition, it has been approved for juvenile idiopathic arthritis, psoriatic arthritis, ankylosing spondylitis, and plaque psoriasis. The drug has had a significant impact in the treatment and management of RA (along with TNFα antagonist MAbs), and it is ranked among the most commercially successful biologics, with 2012 worldwide sales of nearly US$8.4 billion (33).
Subtle binding properties can make a dominating contribution to a molecule’s ultimate activity in a clinical setting, and Fc-fusion proteins and MAbs can differ in this regard. For example, both etanercept and infliximab neutralize soluble TNFα, but only infliximab can induce a clinical response in Crohn’s patients, although both drugs significantly improve RA (34). Direct comparison of the two drugs has shown that only infliximab can bind activated lymphocytes, induce apoptosis, and activate caspase 3 (35). These differences may result from differences in affinity (35) or stoichiometry (25) of the drugs for transmembrane TNFα, which is expressed by activated lymphocytes (35). Scallon et al. suggest that infliximab binds to TNFα with a stoichiometry of 3:1 and that the difference in stoichiomentry may be an important factor in the clinical differences seen between the two drugs (32). Hence, designing optimal binding properties of a receptor for its intended ligand is essential for such a drug to achieve a desired clinical activity.
The clotting factor Fc-fusion protein FIX Fc (Biogen Idec’s Alprolix product) is an enzyme–Fc fusion that was approved in 2014. It is an excellent example of the Fc-fusion protein paradigm used to convert an existing therapy — in this case, a recombinant enzyme limited by its short in vivo half-life — into a second-generation therapy with significantly improved clinical value. FIX plays an essential role in the coagulation cascade. Recombinant FIX was originally licensed in 1997 for treatment of hemophilia B. Because of its relatively short half-life (14–34 hours), frequent repeated intravenous infusions are required for prophylaxis (36).
Developed by Biogen Idec to require less frequent dosing due to an extended half-life, FIX Fc is likely to increase patient compliance and improve clinical outcomes (37). Several characteristics distinguish it from typical Fc-fusion constructs. Only one chain of the Fc dimer is fused to a monomeric FIX molecule, making it a monovalent construct. The monomeric Fc-fusion construct improved the pharmacokinetic and pharmacodynamic profile of FIX even better than a dimeric Fc-fusion construct could (38). FIX is an approximately 55-kDa, vitamin-K– dependent serine protease comprising a number of functional domains that undergo different posttranslational modifications. For that reason, FIX Fc is produced using HEK293 cells — which, unlike CHO cells, can perform all those modifications.
FVIII Fc (Biogen Idec’s Eloctate product) is a second clotting factor Fc that is under development for treatment of hemophilia A. Factor VIII is a 280-kDa glycoprotein with several distinct domains (A1-A2-B- A3-C1-C2). Deletion of its B domain (which contains 19 of 25 potential glycosylation sites) has no impact on function (39), enabling construction of a truncated enzyme. So Biogen Idec developed a recombinant B-domain– deleted factor VIII Fc and found in early work that the homodimer was not well secreted. Generation of the FVIII Fc monomer therefore was enabling to the Fc-fusion technology for this application. Clinical phase 3 testing in patients with severe hemophilia A has been completed (40), and it was approved by the FDA in June 2014.
Fc-Fusion Proteins in Clinical Development
Fc-fusion proteins in clinical development include enzymes, receptor ECDs, and peptides with antagonist or agonist activities.
Also known as AMG386, the peptibody trebananib is a TIE2 mimetic peptide fused to the C-terminus of IgG Fc. It is produced as a homodimer in E. coli. The drug blocks binding of angiopoietin 1 and 2 to the receptor kinase TIE2, inhibiting angiogenesis in ovarian cancer (41). Developed by Amgen, it is currently in phase 3 clinical testing.
A variation on C-terminal fusion peptibodies, blisibimod (A623) consists of four peptides that bind to B-cell activating factor (BAFF) fused to the N-terminus of a human IgG1 Fc. BAFF is a member of the TNF family and is critical to development, maintenance, and survival of B cells. Blisibimod binds to BAFF and inhibits receptor interaction, decreasing B-cell survival (42). Anthera Pharmaceuticals is currently testing the efficacy of this product in a clinical phase 3 trial for treatment of systemic lupus erythematosus.
Dulaglutide is a glucagon-like peptide (GLP)-1 receptor agonist in which a modified GLP-1 peptide is fused to the N-terminus of a modified IgG4 Fc fragment. Developers at Eli Lilly and Company chose to use Fc-fusion IgG4 to minimize effector function (CDC and ADCC) of the fusion construct. They introduced several mutations on the peptide to improve stability and solubility while decreasing immunogenicity. And they found that a flexible linker (GGGGS) between the peptide and the Fc domain improved the product’s in vitro activity (43). Phase 3 clinical testing has been completed for Type 2 diabetes treatment, and a marketing application is pending with the FDA.
Another peptibody platform is Centocor’s Mimetibody technology, in which a bioactive peptide sequence is genetically linked to the N-terminus of an Fc domain. The first example of this approach, CNTO 528 combines a 20– amino-acid erythropoietin mimetic peptide 1 (EMP1) — identified using a combinatorial phage library — with the Fc domain of human IgG1. EMP1 binds to and activates the erythropoietin receptor (44). That construct was replaced by CNTO 530, a second- generation Fc-fusion protein with improved biological and biophysical properties (45). But production challenges have been noted with this class of Fc-fusion molecules (46).
APG 101 is a CD95–IgG1 Fc receptor ECD fusion protein under development by Apogenix. It is a homodimer produced in CHO cells. APG101 blocks the CD95 ligand (CD95L, FasL, Apo-1L) from binding to CD95, reducing cancer-cell migration in malignant glioma and preventing early cell death in myelodysplastic syndrome (47). The product is currently in phase 2 clinical testing.
Novel Fc-Based Scaffolds
The bifunctionality of Fc-fusion proteins can be exploited further. Gillies et al. engineered IL-2–IL-12 and IL-4–GM-CSF bispecific fusions in which the cytokines were positioned in tandem at the C-terminus of Fc (48). In such homodimeric constructs, both cytokine combinations were shown to have synergistic effects as antitumor agents. Moreover, increasing affinity of a binding protein for its target can be achieved using multivalent constructs. Development of heterodimeric Fc platforms based on strand-exchange engineered domains (SEED) — CH3 heterodimers composed of alternating segments of human IgA and IgG CH3 sequences — may allow for the existing homodimeric Fc-fusion platform to extend to including multiple specificities (49).
Multiple avidity has been engineered into Fc fusions to generate both tandem Fc-repeated homodimers (50) and hexameric constructs (51), thus mimicking the structure and therapeutic potential of IgM. A perceived drawback of using polymeric MAbs, particularly in oncology, is that their large size limits tissue penetration. Although it is true that polymers naturally demonstrate slower penetration times, even intact IgM can reach implanted tumors and metastases in patients after intravenous or intraperitoneal administration (52). Slower penetration and accumulation could be an advantage in directing effector function against tumor cells (53).
Approaches that increase valency may be particularly relevant to the growing number of therapeutic antibody fragments entering the clinic: e.g., dromedary VHH minibodies (54), single-chain Fv-based antihuman- immune-deficiency (HIV) proteins (55), or nonantibody-based protein scaffolds that are characterized by smaller size and cysteine-free sequence (56). IgA and IgM also could serve as alternatives to the classic IgG backbone for therapeutic Fc-fusion proteins (57, 58).
Fc-Fusion Proteins as Biosimilars
Fc-fusion proteins are just now becoming candidates for biosimilar development. Because of their higher carbohydrate content than that of MAbs, Fc-fusion protein biosimilars will present additional challenges in achieving analytical biosimilarity (59). First approved in 1998 and now with 16 years on the US market, etanercept is the first such product to become a target for biosimilar development. The patent on etanercept was set to expire in October 2012; however, in November 2011, a new US patent was issued to Roche (licensed exclusively to Amgen) and extended its protection until 2028 (60, 61). Consequently, the introduction of etancerpt biosimilars (62) will occur first in the European Union and markets outside the United States. A strategy to develop a variant of TNFR2-Fc as a potential biobetter also has been reported (63).
Future Perspectives
Fc-fusion proteins are an important and expanding class of biotherapeutics. Following the success of MAbs, engineering efforts have resulted in fusion of one or more effector molecules to IgG Fc. Using this molecular paradigm, effector molecules such as receptor ECDs, cytokines, enzymes, and peptide mimetics of protein- binding domains can be converted into molecular forms with enhanced therapeutic potential. Fc-fusion proteins combine the benefits of a broader repertoire of therapeutic targets with considerable improvements in therapeutic half-life and in vivo stability provided by the interaction with FcRn. Structurally, they are derivatives of MAbs and are manufactured using processes based on the MAb production platform. Fc-fusion proteins are typically homodimers and can be functionally monovalent, bivalent, or multivalent. Newer scaffolds include heterodimers capable of binding to multiple ligands and multimers with increased valency. Of the 46 MAb-based products currently approved by the FDA in the United States, 10 are Fc-fusion proteins. With more in clinical testing and a growing pipeline in preclinical development, the future of this drug class as a path to enable use of biologically active peptides in medicine is bright.
Acknowledgments
The authors acknowledge Laura Shih for her assistance with graphics and have no conflicts of interest in publishing this article herein.
References (Repeated From Part 1)
3 Therapeutic Fc Fusion Proteins. Chamow SM, et al., Eds. Wiley-Blackwell: Hoboken, NJ, 2014.
10 Rath T, et al. Fc-Fusion Proteins and FcRn: Structural Insights for Longer-Lasting and More Effective Therapeutics. Crit. Rev. Biotechnol. 2013: 1–20.
References (New For Part 2)
23 Strober BE, Menon K. Alefacept for the Treatment of Psoriasis and Other Dermatologic Diseases. Dermatol. Ther. 20, 2007: 270–276.
24 Scheinfeld N. Alefacept: Its Safety Profile, Off-Label Uses, and Potential As Part of Combination Therapies for Psoriasis. J. Dermatol. Treat. 18, 2007: 197–208.
25 Linsley PS, et al. Binding Stoichiometry of the Cytotoxic T Lymphocyte-Associated Molecule-4 (CTLA-4): A Disulfide-Linked Homodimer Binds Two CD86 Molecules. J. Biolog. Chem. 270(25) 1995: 15417–15424.
26 Korhonen R, Moilanen E. Abatacept, a Novel CD80/86-CD28 T Cell Co-Stimulation Modulator, in the Treatment of Rheumatoid Arthritis. Bas. Clin. Pharmacol. Toxicol. 104(4) 2009: 276–284.
27 Emamaullee J, et al. Costimulatory Blockade with Belatacept in Clinical and Experimental Transplantation: A Review. Exp. Opin. Biol. Ther. 9(6) 2009: 789–796.
28 Hoffman HM, et al. Long-Term Efficacy and Safety Profile of Rilonacept in the Treatment of Cryopryin-Associated Periodic Syndromes: Results of a 72-Week Open-Label Extension Study. Clin. Ther. 34(10) 2012: 2091–2103.
29 Holash J, et al. VEGF-Trap: A VEGF Blocker with Potent Antitumor Effects. Proc. Nat. Acad. Sci. USA 99(17) 2002: 11393–11398.
30 Cines D, et al. Romiplostim. Nat. Rev. Drug Disc. 7(11) 2008: 887–888.
31 Kuter D, et al. Efficacy of Romiplostim in Patients with Chronic Immune Thrombocytopenic Purpura: A Double-Blind Randomised Controlled Trial. Lancet 371(9610) 2008: 395–403.
32 Scallon B, et al. Binding and Functional Comparisons of Two Types of Tumor Necrosis Factor Antagonists. J. Pharmacol. Exper. Therapeut. 301(2) 2002: 418–426.
33 Hamilton K, Clair EW. Tumour Necrosis Factor-Alpha Blockade: A New Era for Effective Management of Rheumatoid Arthritis. Exp. Opin. Pharmacother. 1(5) 2000: 1041–1052.
34 Sandborn WJ, et al. Etanercept for Active Crohn’s Disease: A Randomized, Double-Blind, Placebo-Controlled Trial. Gastroenterol. 121(5) 2001: 1088–1094.
35 Van den Brande JM, et al. Infliximab but Not Etanercept Induces Apoptosis in Lamina Propria T-Lymphocytes from Patients with Crohn’s Disease. Gastroenterol. 124(7) 2003: 1774–1785.
36 Gater A, et al. Haemophilia B: Impact on Patients and Economic Burden of Disease. Thrombosis Haemostasis 106(3) 2011: 398–404.
37 Powell JS, et al. Safety and Prolonged Activity of Recombinant Factor VIII Fc Fusion Protein in Hemophilia A Patients. Blood 119(13) 2012: 3031–3037. 3
8 Peters RT, et al. Prolonged Activity of Factor IX As a Monomeric Fc Fusion Protein. Blood 115(10) 2010: 2057–2064.
39 Sandberg H, et al. Structural and Functional Characteristics of the B-Domain- Deleted Recombinant Factor VIII Protein, r-VIII SQ. Thrombosis Haemostasis 85(1) 2001: 93–100.
40 Mahlangu J, et al. Phase 3 Study of Recombinant Factor VIII Fc Fusion Protein in Severe Hemophilia A. Blood 123(3) 2014: 317– 325.
41 Eskander RN, Tewari KS. Incorporation of Anti-Angiogenesis Therapy in the Management of Advanced Ovarian Carcinoma: Mechanistics, Review of Phase III Randomized Clinical Trials, and Regulatory Implications. Gynecol. Oncol. 132(2) 2014: 496–505.
42 Stohl W. Therapeutic Targeting of the BAFF/APRIL Axis in Systemic Lupus Erythematosus. Exp. Opin. Therapeut. Targets 18(4) 2014: 473–489.
43 Nauck M, et al. Efficacy and Safety of Dulaglutide Versus Sitagliptin After 52 Weeks in Type 2 Diabetes in a Randomized Controlled Trial (AWARD-5). Diabetes Care 37(8) 2014: 2149–2158.
44 Wrighton NC, et al. Small Peptides As Potent Mimetics of the Protein Hormone Erythropoietin. Science 273(5274) 1996: 458– 464.
45 Sathyanarayana P, et al. CNTO 530 Functions As a Potent EPO Mimetic Via Unique Sustained Effects on Bone Marrow Proerythroblast Pools. Blood 113(20) 2009: 4955–4962.
46 Dorai H, et al. Development of Mammalian Production Cell Lines Expressing CNTO736, a Glucagon Like Peptide-1- MIMETIBODY: Factors that Influence Productivity and Product Quality. Biotechnol. Bioeng. 103(1) 2009: 162–176.
47 Tuettenberg J, et al. Pharmacokinetics, Pharmacodynamics, Safety and Tolerability of APG101, a CD95-Fc Fusion Protein, in Healthy Volunteers and Two Glioma Patients. Internat. Immunopharmacol. 13(1) 2012: 93–100.
48 Gillies SD, et al. Bi-Functional Cytokine Fusion Proteins for Gene Therapy and Antibody-Targeted Treatment of Cancer. Cancer Immunol. Immunother. 51(8) 2002: 449–460. 49 Muda M, et al. Therapeutic Assessment of SEED: A New Engineered Antibody Platform Designed to Generate Mono- and Bispecific Antibodies. PEDS 24(5) 2011: 447–454. 50 Nagashima H, et al. TNF Receptor II Fusion Protein with Tandemly Repeated Fc Domains. J. Biochem. 149(3) 2011: 337–346.
51 Mekhaiel DN, et al. Polymeric Human Fc-Fusion Proteins with Modified Effector Functions. Scientif. Reports 19 October 2011: 124.
52 Kaveri SV, et al. Natural IgM in Immune Equilibrium and Harnessing Their Therapeutic Potential. J. Immunol. 188(3) 2012: 939–945.
53 Peipp M, et al. Effector Mechanisms of Therapeutic Antibodies Against ErbB Receptors. Curr. Opin. Immunol. 20(4) 2008: 436–443.
54 Nelson AL. Antibody Fragments: Hope and Hype. MAbs 2(1) 2010: 77–83.
55 West AP Jr., et al. Single-Chain Fv-Based Anti-HIV Proteins: Potential and Limitations. J. Virol. 86(1) 2012: 195–202.
56 Löfblom J, et al. Non-Immunoglobulin Based Protein Scaffolds. Curr. Opin. Biotechnol. 22(6) 2011: 843–848.
57 Bakema JE, van Egmond M. Immunoglobulin A: A Next Generation of Therapeutic Antibodies? MAbs 3(4) 2011: 352–361.
58 Ammann JU, et al. Detection of Weak Receptor-Ligand Interactions Using IgM and J-Chain-Based Fusion Proteins. Euro. J. Immunol. 42(5) 2012: 1354–1356.
59 Tan Q, et al. Characterization and Comparison of Commercially Available TNF Receptor 2-Fc Fusion Protein Products. MAbs 4(6) 2012: 761–774.
60 Brockhaus M, et al. Human TNF Receptor Fusion Protein. US PTO #8063182, 22 November 2011.
61 Daghlian M. Amgen Issued New Patent on RA Drug Enbrel. The Burrill Report 1 December 2011; www.burrillreport.com/ article-amgen_issued_new_patent_on_ra_ drug_enbrel.html.
62 Maity S, et al. A Non-Innovator Version of Etanercept for Treatment of Arthritis. Biologicals 39(6) 2011: 384–395.
63 Yang T, et al. A Variant of TNFR2-Fc Fusion Protein Exhibits Improved Efficacy in Treating Experimental Rheumatoid Arthritis. PLoS Comput. Biol. 6(2) 2010.
64 Jimenez-Solem, E., et al. Dulaglutide, a long-acting GLP-1 analog fused with an Fc antibody fragment for the potential treatment of type 2 diabetes. Curr. Opin. Molec. Therapeut. 12, 2010: 790–797.
65 Bouman-Thio, E., et al. A Phase I, Single and Fractionated, Ascending-Dose Study Evaluating the Safety, Pharmacokinetics, Pharmacodynamics, and Immunogenicity of an Erythropoietin Mimetic Antibody Fusion Protein (CNTO 528) in Healthy Male Subjects. J. Clin. Pharmacol. 48, 2008: 1197–1207
66 Achuthanandam, R., et al. Pharmacodynamics of CNTO 530 and Darbepoetin Alpha in Human TNF Alpha Transgenic Mice, a Murine Model of Anemia of Chronic Disease. Pharmacol. Pharmacy 2(2), 2001: 17–30
67 Hartmann, N., et al. Recombinant CD95-Fc (APG101) Prevents Graft-Versus-Host Disease in Mice Without Disabling Antitumor Cytotoxicity and T-cell Functions. Blood 121, 2013: 556–565
Angela L. Linderholm is consultant, and corresponding author Steven M. Chamow, PhD, is president and principal consultant of Chamow & Associates, Inc., 747 Laurelwood Drive, San Mateo, CA 94403; 1-650-345- 1878; steve@chamowassociates.com; www.chamowassociates.com