Early in 2021 at the American Society of Clinical Oncology’s (ASCO’s) annual meeting, attendees witnessed the first validation of a novel checkpoint target: lymphocyte activation gene 3 (LAG-3). Bristol Myers Squibb’s (BMS’s) recent success in a phase 3 study of the relatlimab anti-LAG-3 monoclonal antibody (MAb) proved that the combination of LAG-3 and programmed cell death protein 1 (PD-1) is more effective than the standard of care in first-line metastatic melanoma (1). For about seven years, that standard has been the same company’s Opdivo (nivolumab), a PD-1 immune-checkpoint inhibitor (ICI). This marks the first time since the registration of nivolumab and Merck’s anti-PD1 MAb Keytruda (pembrolizumab) that a new ICI has been validated in a phase 3 setting. If these findings are validated further in other applications (e.g., advanced carcinomas), then LAG-3 will become the third immune-checkpoint molecule to be targeted widely in oncology clinics — following cytotoxic T-lymphocyte–associated protein 4 (CTLA-4) and programmed cell death protein and ligand (PD-1–PD-L1) pathways.
Most development in this field is focused on combining two ICIs for anticancer effects. Many such programs are under development, including different PD-1–LAG-3 antagonist combinations (Figure 1). A number of biopharmaceutical companies already are developing anti-LAG-3 MAbs, including Merck, Boehringer Ingelheim, Regeneron, and Novartis. The latter is working on leramilimab (LAG525) licensed from Immutep. The LAG-3 space is likely to become more crowded in the future like that of PD-1, which now is the subject of more than 50 programs going on among several competitors.
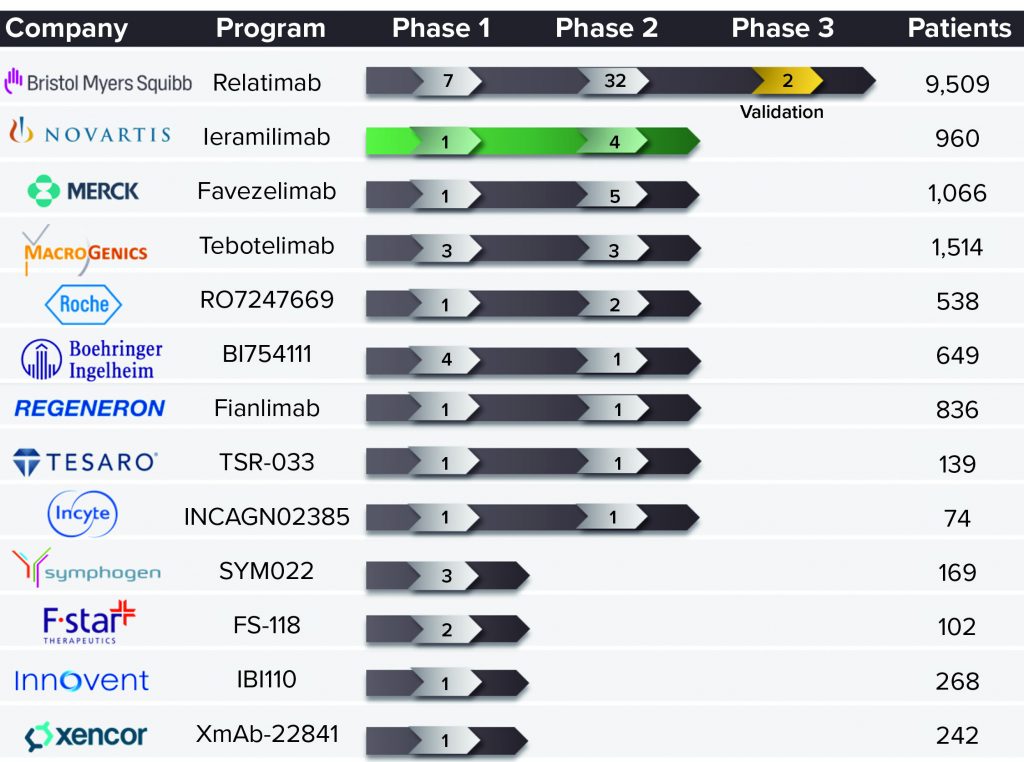
Figure 1: Clinical development of lymphocyte activation gene-3 (LAG-3) blocking
antibodies as of 16 April 2021.
The success of that ICI strategy might be limited, however, considering recent failures of many immunooncology products combined with PD-1 antagonists. For instance, early phase clinical trials combining an indoleamine 2,3-dioxygenase (IDO) inhibitor with a PD-1 inhibitor showed encouraging results. The excitement about the IDO pathway, however, was tempered by negative results in a phase 3 setting. The early days of easily registering PD-1 monotherapies for patients with “hot-tumor” phenotypes —tumor beds infiltrated with many activated T cells producing interferon-γ (IFNγ) — are behind us.
Still, the results of the relatlimab phase 3 trial clearly validate the physiological importance of LAG-3 interaction with its main ligands. Those include major histocompatibility complex (MHC) class II molecules expressed by different antigen-presenting cells (APCs) — e.g., dendritic cells (DCs) and macrophages — at a tumor site or in draining lymph nodes. Simple disruption of that interaction by relatlimab more than doubled patients’ rate of progression-free survival (1).
The success of ICI and related combinations relies primarily on a strong natural antitumor T-cell response to carcinomas with high tumor-cell PD-L1 expressing scores (the hot-tumor phenotype). Just removing the negative regulatory function of CTLA-4, PD-1, or LAG-3 coinhibitory receptors is enough for preactivated T cells to eliminate the tumor cells. Is a therapeutic approach combining two ICIs likely to be effective in more difficult-to-treat populations, namely patients with “cold-tumor” phenotypes and few or no activated T cells in their tumor beds (and with consequently low PD-L1 scores)? Probably not.
That unlikelihood underscores the need for developing alternative approaches that address deficient T-cell priming by boosting or provoking T cells through activation of the APC gene to reinitiate cancer immunosurveillance in poorly immunogenic tumors. It may be more effective mechanistically to combine an APC activator first to boost T cells and ensure that they are present in a tumor bed, then use an ICI such as an anti-PD-1 MAb. In effect, that method would “accelerate the immune system” before combining with another agent to lift the brake on T-cell activity.
That is the approach my company is taking with its lead LAG-3 program. A soluble LAG-3 protein, eftilagimod alpha (efti) activates DCs (the professional APCs) within a few hours of dosage, thus reactivating memory CD4 and CD8 T cells. Such APC activation through MHC II agonism is a proximal event in T-cell priming, so efti could be critical in converting cold tumors to hot tumors, thereby generating effective T-cell immunity. Immutep’s current efforts are focused on using efti in combination with chemotherapy or nonredundant immune modulators such as PD-1 or PD-L1 ICI therapies.
What Is LAG-3?
LAG-3 plays a major role in the immune system by regulating T-cell homeostasis and optimizing immune responses (2–4). The LAG-3 protein is expressed on activated CD4+ and CD8+ effector T cells, on certain regulatory T cells, and on a subset of natural killer (NK) cells (3). As a member of the immunoglobulin (IgG) superfamily with a structure of four extracellular IgG-like domains and a type I transmembrane domain, this protein structurally resembles a CD4 coreceptor. LAG-3’s key interaction is binding to MHC class II with higher affinity than is seen with CD4.
Following T-cell receptor (TCR) engagement on either CD4 or CD8 T cells, LAG-3 receptors are clustered in the immunological synapse, where they negatively regulate signal transduction, leading to a dampening of T-cell proliferation, reduced cytokine production, and ultimately a state of immune tolerance (3, 5, 6). Thus, LAG-3 acts as a coinhibitory receptor like CTLA-4 and PD-1. Continuous exposure of T cells to tumor peptides at a tumor site leads to high sustained expression of LAG-3 T cells, which lose effector function and become exhausted. LAG-3 thereby restricts the ability of those cells to mount an immune response, so blocking LAG-3 can reinvigorate exhausted T cells and boost immune processes (7).
Rationale for Targeting LAG-3–MHC II Interaction in Cancer: Cancer is associated with chronic exposure of a patient’s immune system to tumor antigens, leading to development of dysfunctional or exhausted effector T cells (2). Persistent exposure to tumor antigens causes tumor-infiltrating T cells to express high levels of multiple inhibitory coreceptors such as LAG-3, which ultimately results in functional exhaustion (7). Dysfunctional or exhausted T cells have reduced proliferative capacity. They elicit effector functions (cytotoxicity and cytokine production) upon stimulation through the TCR. LAG-3 is expressed on both CD4+ and CD8+ tumor-infiltrating cells, and blocking the LAG-3 pathway can synergize with antitumor vaccination to improve tumor-specific CD8+ T-cell activation (8).
A naturally circulating soluble form of LAG-3 is known as sLAG-3 (9). Its concentration has been shown to improve survival in patients with breast carcinoma. The amount of sLAG-3 present in human serum represents the level of cell-mediated immunity activation because LAG-3 is produced by activated T cells (10). In 246 breast cancer patients, samples with a high sLAG-3 serum concentration that were taken at the time of first diagnosis predicted longer disease-free duration and overall survival after three and 10 years than those from patients with less sLAG-3 (11). Such results lend strong support to the idea of immunosurveillance playing an active role in restraining tumor growth in cancer, even in advanced disease. Reinforcing cell-mediated natural immunity by targeting the LAG-3–MHC II interaction thus could provide an important advantage over existing therapies.
A Soluble LAG-3 Protein in the Clinic
Stimulatory receptors expressed by APCs include those for two endogenous ligands: CD40 and MHC II. Both receptors are expressed on activated T cells together with receptors for exogenous ligands derived from microbes, the toll-like receptors (TLRs). For activating DCs through a subset of MHC II molecules enriched in lipid rafts (12, 13), Immutep developed a recombinant soluble form of LAG-3 known as the fusion protein LAG-3Ig (efti). Fusing the four extracellular Ig-fold domains of LAG-3 to the Fc of a human IgG1 produces a stable, easily purified (using protein A capture chromatography) recombinant protein that mimics the dimeric structure of the membrane-expressed LAG-3 receptor.
When injected subcutaneously every two weeks at a relatively low dose (30 mg/injection), efti acts as an MHC II agonist, activating DCs and monocytes systemically within a few hours. That leads to reactivation of secondary target cells, memory CD4 and CD8 T cells, and some NK cells. Overall, as a soluble LAG-3 protein targeting DCs, efti leads to a different immunostimulation from that of antagonist LAG-3–specific antibodies that block the LAG-3–MHC II interaction (Figure 2). Cytotoxic T cells are not targeted directly, which causes immune-related adverse reactions with relatlimab, but rather indirectly through a physiological mechanism: APC activation. No additional immune-related adverse reactions were observed even when efti was associated with an anti-PD-1 antibody (14). Also, boosting of systemic innate immunity by efti increases both the number of activated CD8 T cells in circulation and the level of IFN-γ in patient serum, revealing efti’s potential to fight tumor growth through adaptive immunity.
Potential Applications
Because efti acts as a systemic APC activator, it offers three options for use in cancer treatment: in chemoimmunotherapy, in combination immunotherapy, and as an adjuvant. When administered in combination with chemotherapy (chemoimmunotherapy), efti induces APC maturation, facilitating transport of tumor antigenic debris to lymph nodes for presentation to T cells. Efti also can be administered in combination with active immunotherapies, specifically ICIs such as PD-1 or PD-L1 antagonists. In patients who are eligible for anti-PD-1 therapy, adding an APC activator such as efti could help to activate cellular immune-response mechanisms to optimize tumor-cell recognition and killing. That could provide synergistic effects and increase the frequency of durable disease response. Finally, efti can be used as an adjuvant to cancer vaccines or intratumoral injections (in situ immunization). In such cases, it would boost the local immune response to specific tumor antigens.
Chemoimmunotherapy with Efti: Chemotherapy is designed to damage tumor cells, thereby inducing their death, but it also harms healthy cells. A more effective treatment alternative would harness the ability of immune cells to recognize tumor antigens released from dying cells and elicit an effective immune response to suppress the growth of remaining tumor cells (15, 16). Thus, the apoptotic cell death induced by chemotherapy could induce beneficial immunoadjuvant activity in some patients who develop an immune response that targets residual tumor cells (17, 18).
Such beneficial immunoadjuvant activity has been demonstrated in patients under treatment for breast cancer. In one study, patients were treated with a single injection of neoadjuvant paclitaxel chemotherapy to reduce their primary tumors before surgical excision and also to initiate early systemic treatment of distant micrometastases (15). Histological analyses of tumor tissue collected before and after paclitaxel dosing showed extensive lymphocytic infiltration in samples from patients whose tumors had been reduced by the single injection.
Researchers postulate that chemotherapy-induced tumor-specific cytotoxic T lymphocytes can eliminate residual cancer cells and increase patient survival. Another study assessed the lymphocytic infiltrates in breast-cancer tissue after neoadjuvant chemotherapy based on 1,058 core biopsies from two neoadjuvant anthracycline/taxane studies (19). Results showed that the proportion of intratumoral lymphocytes present was a significant and independent predictor of complete disease response (19).
The Active Immunotherapy PAClitaxel (AIPAC) study was designed as a double-blind, placebo-controlled, randomized phase 2b trial to evaluate the safety and efficacy of efti (IMP321) added to weekly paclitaxel as a first-line chemotherapy (compared with paclitaxel and a placebo). The trial involved women with hormone-receptor–positive, metastatic breast carcinoma (MBC) who were ineligible to receive therapy for human epidermal growth factor receptor 2 (HER-2) (20). Administering an APC activator such as efti the day after paclitaxel induced APCs to mature and transport antigenic debris from apoptotic tumor cells to the lymph nodes for presentation to T cells. Encouraging preliminary overall survival and immune-monitoring data were reported at the virtual San Antonio Breast Cancer Symposium in December 2020 (21).
Efti Combined with an ICI: Several inhibitors of PD-1–PD-L1 interaction have been developed as treatments for different indications. Generally, the response rate to single agents used in a first-line metastatic setting is low: 12% for ovarian and breast cancers and 20% for refractory/metastatic head and neck squamous cell carcinoma (HNSCC). The same is true for second-line treatment of non–small-cell lung cancer (20%) (22–27). Treatment tumor biopsies have shown that nonresponding patients either have few tumor-infiltrating lymphocytes in the tumor bed or exhibit a nonfunctional immune response (no intratumoral IFN-γ and PD-1/PD-L1 negative tumor-infiltrating lymphocytes) (27). Understanding the reasons why many patients do not respond to PD-1–PD-L1 blockade is key in developing new treatment combinations in this field.
LAG-3 is coexpressed with PD-1 on tumor-infiltrating lymphocytes in a number of human tumors (3), and it has been identified as a potential checkpoint for tumor escape during PD-1–PD-L1 inhibitor therapy (29). The mainstream double-ICI approach (anti-LAG-3 with anti-PD-1 treatment) is intended for one subset of patients with >50% PD-L1 expression (hot tumors). Combining efti with an ICI, however, is a unique and more comprehensive approach. As an APC activator, efti indirectly activates T cells through a physiological mechanism, enabling more patients with all levels of PD-L1 expression to respond and benefit from treatment. In patients who are eligible for anti-PD-1 therapy, the addition of an APC activator could help to activate their cellular immune-response mechanisms and optimize tumor-cell recognition and killing. Through these synergistic effects, we might achieve a higher frequency of deep and durable disease responses with no additional or substantial toxicity.
Results from testing in cancer patients show that efti helps to sustain and proliferate tumor-antigen–specific CD8+ T cells and peripheral blood mononuclear cells (PBMCs), thus demonstrating its ability to boost the CD8+ T-cell memory response ex vivo (30). When the APCs of those PBMCs were exposed to efti and peptide antigens ex vivo for two days, their phenotype and resulting cytokines/chemokines indicated that the LAG-3-mediated effect depends on a direct activation of circulating APCs.
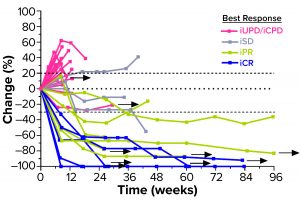
Figure 3: Second-line head-and-neck squamous cell carcinoma (HNSCC) patients’ responses to a combination of efti and pembrolizumab (PD-1 antagonist); arrows indicate patients who remain in treatment (30). iUPD = unconfirmed progression; iCPD = confirmed progression; iSD = stable disease; iPR = partial response; iCR = complete response.
A series of Two-ACTive Immunotherapy (TACTI) trials is evaluating the effect of combining efti with pembrolizumab (a PD-1 antagonist) in different indications such as metastatic melanoma, NSCLC, and HNSCC (14). In the ongoing TACTI-002 study, for example, hard-to-treat second-line patients with metastatic HNSCC who were not preselected for PD-L1 expression on their tumor cells received 30 mg of efti subcutaneously every two weeks in combination with standard-of-care pembrolizumab. As of April 2021, those patients showed a durably high response rate (35% for evaluable patients) (Figure 3).
Efti As an Adjuvant to a Cancer Vaccine: Cancer-vaccine–induced T-cell responses depend largely on the vaccine’s potency to activate, mature, and migrate DCs to draining lymph nodes for activation of antigen-specific effector T cells. Thus, state-of-the-art DC inducers are key components of therapeutic vaccines. This has been demonstrated in mice: Subcutaneous or intramuscular coinjection of LAG-3 Ig and antigens (irradiated tumor cells, protein antigens, or DNA plasmids) ultimately inhibits tumor growth (32–35).
When used as a low-dose adjuvant to a vaccine, efti boosts the local immune system reaction through APC activation and increases presentation of antigenic peptides to T cells. Kano et al. reported proof of efficacy when combining efti with the TLR-3 agonist polyinosinic-polycytidylic acid and a tumor-antigen–peptide T-cell vaccine in a murine mastocytoma model (36). Improved T-cell infiltration was a key feature of tumor control when using efti as an adjuvant to a cancer vaccine, currently the subject of several ongoing phase 1–2 trials (37).
In a similar approach for the INSIGHT trial, efti is injected directly into a tumor mass (the antigen source), a therapeutic method called “in situ immunization” (38).
First-in-Class Potential
With promising clinical results continuing to emerge in immunooncology, the potential of efti as a first-in-class MHC II agonist combined with different standards of care is only beginning to be uncovered. Our next development steps will lead to registration-relevant trials, including phase 3 studies for certain indications. Process-development activities at commercial scale are ongoing in parallel.
References
1 Lipson EJ, et al. Relatlimab (RELA) Plus Nivolumab (NIVO) Versus NIVO in First-Line Advanced Melanoma: Primary Phase III Results from RELATIVITY-047 (CA224-047). J. Clin. Oncol. 39(15) 2021: 9503; https://doi.org/10.1200/JCO.2021.39.15_suppl.9503.
2 Anderson AC, Joller N, Kuchroo VK. Lag-3, Tim-3, and TIGIT: Co-Inhibitory Receptors with Specialized Functions in Immune Regulation. Immunity 44(5) 2016: 989–1004; https://doi.org/10.1016/j.immuni.2016.05.001.
3 Joller N, Kuchroo VK. Tim-3, Lag-3, and TIGIT. Curr. Top. Microbiol. Immunol. 410, 2017: 127–156; https://doi.org/10.1007/82_2017_62.
4 Shan C, Li X, Zhang J. Progress of Immune Checkpoint LAG-3 in Immunotherapy. Oncol. Lett. 20(5) 2020: 207; https://doi.org/10.3892%2Fol.2020.12070.
5 Hannier S, et al. CD3/TCR Complex-Associated Lymphocyte Activation Gene-3 Molecules Inhibit CD3/TCR Signaling. J. Immunol. 161(8) 1998: 4058–4065; https://www.jimmunol.org/content/161/8/4058.long.
6 Long L, et al. The Promising Immune Checkpoint LAG-3: From Tumor Microenvironment to Cancer Immunotherapy. Genes Cancer 9(5–6) 2018: 176–189; https://doi.org/10.18632/genesandcancer.180.
7 Maruhashi T, et al. LAG-3: From Molecular Functions to Clinical Applications. J. Immunother. Cancer 8(2) 2020: e001014; https://doi.org/10.1136/jitc-2020-001014.
8 Grosso JF, et al. LAG-3 Regulates CD8+ T Cell Accumulation and Effector Function in Murine Self- and Tumor-Tolerance Systems. J. Clin. Invest. 117(11) 2007: 3383–3392; https://doi.org/10.1172/jci31184.
9 Triebel F. LAG-3: A Regulator of T-Cell and DC Responses and Its Use in Therapeutic Vaccination. Trends Immunol. 24(12) 2003: 619–622; https://doi.org/10.1016/j.it.2003.10.001.
10 Triebel F, et al. LAG-3, a Novel Lymphocyte Activation Gene Closely Related to CD4. J. Exp. Med. 171(5) 1990: 1393–1405; https://doi.org/10.1084/jem.171.5.1393.
11 Triebel F, Hacene K, Pichon MF. A Soluble Lymphocyte Activation Gene-3 (sLAG-3) Protein As a Prognostic Factor in Human Breast Cancer Expressing Estrogen or Progesterone Receptors. Cancer Lett. 235(1) 2006: 147–153; https://doi.org/10.1016/j.canlet.2005.04.015.
12 Andreae S, et al. Maturation and Activation of Dendritic Cells Induced By Lymphocyte Activation Gene-3 (CD223). J. Immunol. 168(8) 2002: 3874–3880; https://doi.org/10.4049/jimmunol.168.8.3874.
13 Andreae S, Buisson S, Triebel F. MHC Class II Signal Transduction in Human Dendritic Cells Induced By a Natural Ligand, the LAG-3 Protein (CD223). Blood 102(6) 2003: 2130–2137; https://doi.org/10.1182/blood-2003-01-0273.
14 Atkinson V, et al. Eftilagimod Alpha, a Soluble Lymphocyte Activation Gene-3 (LAG-3) Protein Plus Pembrolizumab in Patients with Metastatic Melanoma. J. Immunother. Cancer 8(2) 2020: e001681; https://doi.org/10.1136/jitc-2020-001681.
15 Demaria S, et al. Development of Tumor-Infiltrating Lymphocytes in Breast Cancer after Neoadjuvant Paclitaxel Chemotherapy. Clin. Cancer. Res. 7(10) 2001: 3025–3030;
https://clincancerres.aacrjournals.org/content/7/10/3025.long.
16 Casares N, et al. Caspase-Dependent Immunogenicity of Doxorubicin-Induced Tumor Cell Death. J. Exp. Med. 202(12) 2005: 1691–1701; https://doi.org/10.1084/jem.20050915.
17 Nistico P, et al. Chemotherapy Enhances Vaccine-Induced Antitumor Immunity in Melanoma Patients. Int. J. Cancer 124(1) 2009: 130–139; https://doi.org/10.1002/ijc.23886.
18 Apetoh L, et al. Toll-Like Receptor 4-Dependent Contribution of the Immune System to Anticancer Chemotherapy and Radiotherapy. Nat. Med. 13(9) 2007: 1050–1059; https://doi.org/10.1038/nm1622.
19 Denkert C, et al. Tumor-Associated Lymphocytes As an Independent Predictor of Response to Neoadjuvant Chemotherapy in Breast Cancer. J. Clin. Oncol. 28(1) 2010: 105–113; https://doi.org/10.1200/jco.2009.23.7370.
20 Dirix L, Triebel F. AIPAC: A Phase IIb Study of Eftilagimod Alpha (IMP321 or LAG-3Ig) Added to Weekly Paclitaxel in Patients with Metastatic Breast Cancer. Future Oncol. 15(17) 2019: 1963–1973; https://doi.org/10.2217/fon-2018-0807.
21 Wildiers H, et al. Abstract PD14-08: Primary Efficacy Results from AIPAC: A Double-Blinded, Placebo Controlled, Randomized Multinational Phase IIb Trial Comparing Weekly Paclitaxel Plus Eftilagimod Alpha (Soluble LAG-3 Protein) Vs. Weekly Paclitaxel Plus Placebo in HR-Positive Metastatic Breast Cancer Patients. Cancer Res. 81(4S) 2021: PD14-08-PD14-08; https://doi.org/10.1158/1538-7445.SABCS20-PD14-08.
22 Pujade-Lauraine E, et al. Bevacizumab Combined with Chemotherapy for Platinum-Resistant Recurrent Ovarian Cancer: The AURELIA Open-Label Randomized Phase III Trial. J. Clin. Oncol. 32(13) 2014: 1302–1308; https://doi.org/10.1200/jco.2013.51.4489.
23 Cohen EE, et al. Pembrolizumab (Pembro) Vs. Standard of Care (SOC) for Recurrent or Metastatic Head and Neck Squamous Cell Carcinoma (R/M HNSCC): Phase 3 KEYNOTE-040 Trial. Annals Oncol. 28(S5) 2017: v605–v649; https://doi.org/10.1093/annonc/mdx440.
24 Herbst RS, et al. Pembrolizumab Versus Docetaxel for Previously Treated, PD-L1-Positive, Advanced Non-Small-Cell Lung Cancer (KEYNOTE-010): A Randomised Controlled Trial. Lancet 387(10027) 2016: 1540–1550; https://doi.org/10.1016/s0140-6736(15)01281-7.
25 Somasundaram A, Burns TF. Pembrolizumab in the Treatment of Metastatic Non-Small-Cell Lung Cancer: Patient Selection and Perspectives. Lung Cancer 8, 2017: 1–11; https://doi.org/10.2147/lctt.s105678.
26 Bauml J, et al. Pembrolizumab for Platinum- and Cetuximab-Refractory Head and Neck Cancer: Results from a Single-Arm, Phase II Study. J. Clin. Oncol. 35(14) 2017: 1542–1549; https://doi.org/10.1200/jco.2016.70.1524.
27 Varga A, et al. Antitumor Activity and Safety of Pembrolizumab in Patients (pts) with PD-L1 Positive Advanced Ovarian Cancer: Interim Results from a Phase Ib Study. J. Clin. Oncol. 33(15S) 2015: 5510; https://doi.org/10.1200/jco.2015.33.15_suppl.5510.
28 Herbst RS, et al. Predictive Correlates of Response to the Anti-PD-L1 Antibody MPDL3280A in Cancer Patients. Nature 515(7528) 2014: 563–567; https://doi.org/10.1038/nature14011.
29 McCusker MG, Orkoulas-Razis D, Mehra R. Potential of Pembrolizumab in Metastatic or Recurrent Head and Neck Cancer: Evidence to Date. Onco. Targets Ther. 13, 2020: 3047–3059; https://doi.org/10.2147/ott.s196252.
30 Casati C, et al. Soluble Human LAG-3 Molecule Amplifies the In Vitro Generation of Type 1 Tumor-Specific Immunity. Cancer Res. 66(8) 2006: 4450–4460; https://doi.org/10.1158/0008-5472.can-05-2728.
31 Brana I, et al. Results from a Phase II Study of Eftilagimod Alpha (Soluble LAG-3 Protein) and Pembrolizumab in Patients with PD-L1 Unselected Metastatic Second-Line Squamous Head and Neck Carcinoma. J. Clin. Oncol. 39(15S) 2021: 6028–6028; https://doi.org/10.1200/JCO.2021.39.15_suppl.6028.
32 Prigent P, et al. LAG-3 Induces Tumor Regression and Antitumor Immune Responses In Vivo. Eur. J. Immunol. 29(12) 1999: 3867–3876; https://doi.org/10.1002/(sici)1521-4141(199912)29:12%3C3867::aid-immu3867%3E3.0.co;2-e.
33 El Mir S, Triebel F. A Soluble LAG-3 Molecule Used As a Vaccine Adjuvant Elicits Greater Humoral and Cellular Immune Responses to Both Particulate and Soluble Antigens. J. Immunol. 164(11) 2000: 5583–5589; https://doi.org/10.4049/jimmunol.164.11.5583.
34 Cappello P, et al. LAG-3 Enables DNA Vaccination to Persistently Prevent Mammary Carcinogenesis in HER-2/Neu Transgenic BALB/c Mice. Cancer Res. 63(10) 2003: 2518–2525; https://cancerres.aacrjournals.org/content/63/10/2518.long.
35 Di Carlo E, et al. Immunological Mechanisms Elicited at the Tumour Site By Lymphocyte Activation Gene-3 (LAG-3) Versus IL-12: Sharing a Common Th1 Anti-Tumour Immune Pathway. J. Pathol. 205(1) 2005: 82–91; https://doi.org/10.1002/path.1679.
36 Kano Y, et al. Combined Adjuvants of Poly(I:C) Plus LAG-3-Ig Improve Antitumor Effects of Tumor-Specific T Cells, Preventing Their Exhaustion. Cancer Sci. 107(4) 2016: 398–406; https://doi.org/10.1111/cas.12861.
37 Nakajima M, et al. A Phase I Study of Multi-HLA-Binding Peptides Derived from Heat Shock Protein 70/Glypican-3 and a Novel Combination Adjuvant of hLAG-3Ig and Poly-ICLC for Patients with Metastatic Gastrointestinal Cancers: YNP01 Trial. Cancer Immunol. Immunother. 69(8) 2020: 1651–1662; https://doi.org/10.1007/s00262-020-02518-7.
38 Mueller DW, et al. The “INSIGHT” Trial: An Explorative, Open-Labeled Phase I Study to Evaluate the Feasibility and Safety of Intra-Tumoral, Intra-Peritoneal, and Subcutaneous Injections with IMP321 (LAG-3Ig Fusion Protein) for Advanced Stage Solid Tumor Entities. J. Clin. Oncol. 36(15) 2018: supplement; https://doi.org/10.1200/JCO.2018.36.15_suppl.TPS3129.
Frédéric Triebel, MD, PhD, is chief scientific officer and chief medical officer
at Immutep, 21 rue Jean Rostand, 91893 Orsay, France; 33-6609-16593;
https://www.immutep.com.