Aggregation is a common cause of protein instability, which renders a biologic product unfit for therapeutic use. Sometimes it is difficult to purify monomeric proteins from oligomers because of similarities in their isoelectric points (pIs). Proteins such as hormones have pI ranges similar to their oligomers and thus can be difficult to separate out using a conventional polishing chromatographic step such as ion exchange. With those pI similarities, removal of oligomers to a considerable extent by ion exchangers can compromise protein recovery, thus causing unacceptable product loss during processing.
To minimize the yield loss during extensive reduction of oligomers, we considered the use of novel techniques with process optimization. A downstream process should be robust and reproducible at scale-up with minimum impact on recovery. Much research is ongoing in this area, with limited successes in purifying proteins from oligomers. Thus, techniques are needed that can reduce oligomers to well below acceptance limits and ensure optimal safety levels. Protein aggregation can be reversible or irreversible because the oligomers formed may be soluble or insoluble, covalent or noncovalent, and native or nonnative. Depending on the physicochemical characteristics of a protein, oligomers can compromise product quality — specifically in safety, efficacy, delivery, dosing, and/or marketability.
Protein aggregation can be initiated by a number of physical factors, including temperature (e.g., freezing and thawing), ionic strength, and interacting microenvironment exposure in a bioprocess (e.g., mixing during a change in pH or temperature). Chemical aggregation pathways include disulfide-bond formation or exchange due to oxidation, deamidation, and formation of free thiol groups. Sometimes, highly concentrated liquid formulations are required to achieve a desired therapeutic dose. Proteins in such drug products can develop aggregation as high concentrations promote associations among them, ultimately decreasing drug stability and compromising shelf life. Most pharmacopoeias specify oligomer content in a range from 0.5% to 3.0% depending on the nature and origin of a therapeutic protein as well as duration of therapy.
For biotherapeutics, oligomer presence not only is undesirable, but also introduces the risk of immunogenic reactions upon injection. Protein molecules experience ranges of pH, ionic strength, diafilterability, and concentration factors in downstream processing, all of which can influence the degree of oligomer formation. Size-exclusion chromatography (SEC) is an efficient unit operation for removing oligomers, but it is not used widely because of low resolution, sample-volume limitations, long process times, and high costs.
Ion-exchange chromatography (IEC) can be useful for oligomer-content reduction at production scale both in binding and nonbinding modes, depending on the type of ion-exchange resin used. A working pH that is closer to a protein’s pI gives the molecule near-neutral charge. Oligomers generally carry charges that are more positive or negative than that of the monomer at such pH levels. Technologies such as anion-exchange membrane absorbers are becoming attractive because of their high throughput and binding capacities as well as cost-effectiveness. Also, additional steps of buffer exchange can be averted with introduction of salt-tolerant membranes.
Multimodal or mixed-mode chromatography (MMC) is another widely used technique for removal of oligomers from protein product streams. With increased selectivity and a broader pH working range, MMC is preferable to use over IEC and hydrophobic-interaction chromatography (HIC) methods. The ligands of mixed-mode resins typically contain a combination of binding sites for ion exchange, hydrogen bonding, and hydrophobic groups, which provides for different protein–ligand interactions. In MMC process development, however, process engineers should consider the compatibility of protein and buffer solvents with resins, columns, and process equipment.
Objective
Bharat Serums and Vaccines Limited (BSV) in India evaluated a number of chromatographic resins for oligomer removal from a recombinant human therapeutic protein hormone used for infertility treatment. During the purification process of this recombinant drug substance, the protein was concentrated with pharmacopeial specification of ≤0.8 mg/L, and an oligomer content of >1% was recorded for the drug substance.
Our initial experiments used different anion-exchange resins chosen on the basis of protein knowledge accumulated during research and development. We also evaluated membrane chromatography and MMC resins as options for oligomer reduction. To study the reduction in oligomer content (if any) and protein recovery, we characterized elution samples from each purification technique using analytical procedures such as SEC high-performance liquid chromatography (SEC-HPLC) and reducing or nonreducing sodium-dodecyl sulfate polyacrylamide gel electrophoresis (SDS-PAGE). Buffer conditions and loading strategies were based on protein properties and previous experience.
Methodology and Framework
Oligomer Reduction Using Ion-Exchange Resins: We evaluated two different quaternary ammonium ion-exchange resins for oligomer reduction with this protein: IEC resin 1 (Toyopearl GigaCap Q-650M from Tosoh Bioscience) and IEC resin 2 (Capto Q from Cytiva, formerly known as GE Healthcare), both in binding and nonbinding (flow-through) modes. Preload material for both IEC resins 1 and 2 in nonbinding mode was a sodium phosphate buffer system at pH 8.0 with 30-mS/cm conductivity; in addition for IEC resin 2, we used a sodium phosphate buffer at pH 6.5 with conductivities of 10 mS/cm and 30Â mS/Â cm in separate trials. Preload material in binding mode for both resins was in sodium phosphate buffer at pH 6.5 with a conductivity of 10 mS/cm.
To elute the protein in binding mode, we used a step gradient with increasing conductivity from 10 to 50 mS/cm. Preload protein concentration was 0.1 mg/mL for initial processes, then 0.8 mg/mL and 1 mg/mL for later processes. A 4-mL resin volume was packed in an XK 16/20 column (Cytiva) with a bed height of 2 cm for all these experiments. Clean-in-place (CIP) was performed before and after processing with 5 column volumes (CV) of 0.5 M NaOH solution at a suitable flowrate with a residence time ≥4 minutes. We loaded 20 mL of protein material onto the column at suitable flowrate with residence time ≥4 minutes to maintain the ratio of 5:1 for preload material per gel volume.
Oligomer Reduction Using Membrane Chromatography: We used two membrane chromatographic techniques: one with a salt-tolerant, primary-amine, anion-exchange membrane adsorber of 1-mL volume (Sartobind STIC PA from Sartorius Stedim Biotech) and the other a hydrophobic membrane adsorber of 3-mL volume (Sartobind Phenyl, also from Sartorius Stedim Biotech). Preload material was in sodium phosphate buffer at pH 7.5 with 30 mS/cm conductivity. Because of the membrane adsorbers’ high binding capacities, we loaded 25 mL onto each membrane with a ratio of 25:1 preload material to membrane volume at the operating flow rate of 2 mL/min. An elution sample from the initial trial run was collected in 5-mL fractions to a test pool that we analyzed for protein concentration and oligomer content using SEC.
Oligomer Reduction Using MMC Resins: We evaluated three different mixed-mode resins: MMC resin 1 (Capto Adhere from Cytiva), MMC resin 2 (Nuvia aPrime 4A from Bio-Rad Laboratories), and MMC resin 3 (Toyopearl NH2-750F from Tosoh Bioscience). Preload material in these experiments was in sodium phosphate buffer at pH 7.5 with 30-mS/cm conductivity. Here we used ~0.8-mg/mL protein solutions for the initial trial runs.
For later processes, we used concentrated protein solutions of ≤1.5 mg/mL with the same buffer conditions. CIP was performed before and after processing with 5 CV of 0.5 M NaOH solution. Based on results of the previous experiments, we changed the protein-loading volume ratio to 15:1 per resin volume at a 4-min residence time. A resin volume of 4 mL was packed in the XK 16/20 column with a bed height of 2 cm for initial studies, followed by a resin volume of 10 mL (bed height 5 cm) for later tests using equilibration buffer conditions matching those of the preload material. We used a step gradient of increasing conductivity from 35 mS/cm to 50 mS/cm for this experimental setup. All elute samples were analyzed for oligomer content.
Results and Discussion
Oligomer Reduction Using Ion-Exchange Resins: Anion-exchange resins were chosen for these studies on the basis of protein chemistry. Table 1 lists protein-recovery data from oligomer reduction using the anion-exchange resins.
IEC resin 1 shows comparatively low yield, with little oligomer reduction, so we discontinued evaluation of it. IEC resin 2 showed higher oligomer-content reduction (~84%) in binding mode, so further experimentations were carried out with increasing ionic concentrations in nonbinding mode (flow-through), with yields that were ~20% less. We found that higher volumes of buffer (20–30 CV) were required for elution of protein, resulting in protein dilution and decreased protein concentration. Based on these limitations, we carried out no further experiments using IEC resins.
Oligomer Reduction Using Membrane Adsorbers: Protein was loaded on membrane adsorbers at 0.8 mg/mL during this experiment under nonbinding (flow-through) conditions. Table 2 lists oligomer-reduction and protein-recovery data using membrane adsorbers. Both membranes exhibited much less reduction in oligomer content than other options but showed high protein recoveries. Because of the lower oligomer-removing capacities, the given membrane adsorbers were not subjected to further study.
Oligomer Reduction Using MMC Resins: Based on earlier experiments using IEC resins and membrane adsorbers, we selected for MMC resin studies a sodium phosphate buffer at pH 7.50 with 30-mS/cm conductivity. These resins were evaluated under nonbinding (flow-through) conditions. Table 3 lists oligomer-reduction and protein-recovery data for the MMC resins.
MMC resin 1 consistently showed higher oligomer reduction of >85% with process yields of ≥95%. MMC resins 2 and 3 exhibited comparable protein recoveries but much lower oligomer reduction, so we did not consider them for further study. We further evaluated MMC resin 1 by changing the ratio of preload to resin volumes to evaluate its effect on oligomer removal. Table 4 lists the oligomer-removal results.
Using a 5:1 ratio of preload to resin volume provided the highest oligomer reduction with complete protein recovery. The experiment with a 25:1 ratio of preload to resin volume accounted for lower oligomer reduction; a 15:1 ratio gave results that were comparable with the 5:1 ratio experiment. Hence, we finalized the 15:1 parameter for linearity during scale-up and to check reproducibility in further studies by keeping other parameters the same. Table 5 lists final oligomer-removal data for MMC resin 1.
Based on those results, we found oligomer-content reduction and protein recovery to be reproducible. So we finalized a process based on MMC resin 1 for oligomer-content reduction in our final purification scheme using preload material at a concentration of 1.0–1.2 mg/mL. That was adjusted to equilibration with a sodium phosphate buffer at pH 7.50 and 30-mS/cm conductivity. The ratio of loading to resin volume was finalized at 15:1. Elution is carried out in flow-through (nonbinding) mode with 15 CV of above-mentioned buffer at five minutes residence time. CIP of resin bed before and after processing is carried out using 0.5 M NaOH for 5 CV of packed resin volume.
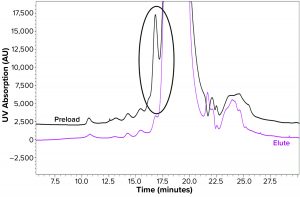
Figure 1: Size-exclusion chromatogram shows reduction in oligomer content (highlighted in circle).
Final Choice
We evaluated the capabilities of different resins and membranes for minimizing oligomer content in final drug substance of a recombinant hormone. MMC resin 1 (Capto Adhere from Cytiva) provided satisfactory results with ~85–95% reduction in oligomer content and good protein recovery of >95%. That became evident when we compared the oligomer-reduction data from pre- and postelute samples of the resin. The latter showed higher removal of oligomers (Figure 1).
This MMC step yields an oligomer content in the range of 0.2–0.3% for BSV’s recombinant-hormone drug substance, which passes our release specification allowable range of 0.5–3.0% (based on pharmacopoeial recommendations for the protein). Introducing MMC contributes to the quality aspect of our final drug substance, with minimal product loss in the final purification process. The high oligomer-binding capacity of this resin makes it a cost-effective option for low-volume acquisition. The mixed-mode step has been scaled up at our manufacturing site for the recombinant hormone with similar results in oligomer reduction and protein recovery (data not shown). Thus, it would be recommended to use MMC resin for effective oligomer reduction. The results reported herein apply only to the protein product studied and would be expected to differ for other protein products.
Further Reading
Fink AL. Protein Aggregation: Folding Aggregates, Inclusion Bodies and Amyloid. Fold Des. 3(1) 1998: R9–R23; https://doi.org/10.1016/s1359-0278(98)00002-9.
Fradkin AH, Carpenter JF, Randolph TW. Immunogenicity of Aggregates of Recombinant Human Growth Hormone in Mouse Models. J. Pharm. Sci. 98(9) 2009: 3247–3264; https://doi.org/10.1002/jps.21834.
ICH Q6B. Test Procedures and Acceptance Criteria for Biotechnological/Biological Products. US Fed. Reg. 64, 1 August 1999: 44928; https://database.ich.org/sites/default/files/Q6B%20Guideline.pdf.
Product Specifications: Capto Adhere Multimodal Chromatography Resin. Cytiva: Marlborough, MA, 2020; https://www.cytivalifesciences.com/en/in/shop/chromatography/resins/multimodal/capto-adhere-multimodal-chromatography-resin-p-05643#tech-spec-table.
Roberts CJ. Protein Aggregation and Its Impact on Product Quality. Curr. Opin. Biotechnol. 30, December 2014: 211–217; https://doi.org/10.1016/j.copbio.2014.08.001.
Yigzaw Y, et al. Ion Exchange Chromatography of Proteins and Clearance of Aggregates. Curr. Pharm. Biotech. 10(4) 2009: 421–426; https://doi.org/10.2174/138920109788488842.
Corresponding author Pranav Milind Gupte is assistant manager, Mahesh Gavasane is general manager, Tushar Bhabal is executive, and Saher Cheulkar is officer of biotech R&D in recombinant biotherapeutics downstream processing and formulation development at Bharat Serums and Vaccines Limited, Third Floor, Liberty Tower, Plot No. K-10, Behind Reliable Plaza, Kalwa Industrial Estate, Airoli, Navi Mumbai – 400 708, India; 022-45043100; pranav.gupte@bharatserums.com.
ÄKTA Prime Plus, Capto Adhere, Capto Q, and XK 16/20 are registered trademarks of Cytiva (formerly GE Healthcare). Toyopearl GigaCap Q-650M and Toyopearl NH2-750F are registered trademarks of Tosoh Corporation. Nuvia aPrime 4A is a registered trademark of Bio-Rad Laboratories, Inc. Sartobind STIC PA and Sartobind Phenyl are registered trademarks of Sartorius Stedim Biotech.