Blow–fill–seal (BFS) technology has been recognized by the industry as an advanced aseptic solution (1–3). Catalent Pharma Solutions has been commercially supplying sterile BFS products to the pharmaceutical industry for decades, primarily in the respiratory and topical ophthalmic markets. Such product formulations range from simple solutions to emulsions with drug substances from classical small molecules to large complex proteins such as biologics. The company also has optimized BFS processes and its Advasept plastic container system for the manufacture of sterile injectable products.
The Advasept container system provides significant advantages over traditional, commercial, glass container systems for the parenteral/injectable market space. Benefits include reduced container breakage and an absence of glass delamination (4). The automated aseptic filling design of BFS advanced aseptic technology drastically reduces the risks associated with traditional aseptic manufacturing. BFS forms, fills, and seals vial contents in less than 15 seconds within a closed, class A environment, by which it reduces the risk of contamination from both microbial and foreign particulate sources.
Advasept technology provides customized mold designs that support anticounterfeiting measures, which is particularly advantageous for expensive biologics. Customized mold designs can reduce vial headspace, which can help improve protein stability by lessening product agitation during shipping. Agitation with air has been shown to cause some protein aggregation and precipitation in solution.
Potential compatibility concerns do exist with BFS-processed container systems for biologics. For example, the molded plastic reaches elevated temperatures during filling, which may compromise the stability of biologic formulations and could lead to potential leachables (5–11) from BFS-processed plastic container systems, which also could affect biologic stability and safety of the formulation. In addition, elevated gas permeation through plastic containers might affect the stability of oxygen-sensitive biologics. All these effects need to be considered during initial container compatibility screening. Catalent has worked to optimize its molded-plastic process to reduce solution temperatures (for 0.5-mL fills) to near 40 °C at the time of product filling. The company’s assumption is that the temperature of a contained solution would fall steadily after units come out of the filling suite and are packaged under ambient conditions (data not provided).
To address those potential compatibility concerns, we conducted a comprehensive study to evaluate the influence of our optimized BFS process and container system on a model monoclonal antibody (MAb) formulation. Table 1 lists the formulation of this model MAb (molecular weight 166 kDa). We used glass vials with uncoated stoppers as controls for this study. Several analytical methods helped us evaluate MAb stability and leachables from the container system (Table 2).
Materials and Methods
We filled the model MAb formulation both in glass and the optimized Advasept BFS container system using a qualified laminar-flow hood to minimize microbial contamination. The MAb formulation was filtered by a 0.2-µm Nalgene filter unit and filled in glass vials into which uncoated stoppers were placed. In parallel, it was filtered into a sterile bag and shipped to Catalent’s BFS manufacturing facility, where the formulation was aseptically transferred to BFS Advasept stoppered vials. Samples were stored at 5 °C and tested at a series of time intervals using methods described in Table 2.
Results
pH: We found no apparent pH change for either glass or BFS-stoppered vials when testing both before and after filling, as well as upon nine months’ storage at 5 °C. Results were within the target range (data not shown).
Appearance: We observed no visible foreign particles for either glass or BFS-stoppered vials when testing both before and after filling, as well as upon nine months of storage at 5 °C. Results were within the target range (data not shown).
Potency: We used ultraviolet absorption (280 nm) and an activity assay to examine potency of the MAb before and after filling, as well as after nine months of storage at 5 °C (Table 1). The UV data indicate no apparent change in potency for both vial formats when tested before and after filling, and after nine months of storage at 5 °C both were within target range.
We also determined potency using a responsive cell line in a complement- dependent cytotoxic assay with a fluorescence read-out. Figure 1 compares a dilution series generated with a standard, the MAb formulation in glass, and the same formulation in an Advasept vial. These activity data show comparable potency values for the formulations in glass and Advasept vials after nine months of storage at 5 °C (all data not included).
Stability: We used five different methods of testing product stability (Table 2). These include size-exclusion chromatographic and nanoparticle tracking analysis, sodium-dodecyl sulfate polyacrylamide gel electrophoresis (SDS-PAGE), capillary isoelectric focusing, and peptide mapping by ultraperformance liquid chromatography with UV and mass- spectrometric detection.
SEC Analysis: All SEC data (pre- and postfill, and upon storage) show comparable percentages of high–molecular-weight (HMW) species for both the glass and Advasept vials (Figures 2–4).
NTA Analysis: As Table 3 shows, we observed slightly larger aggregates in the Advasept vials than in glass. However, the total number of particles are statistically comparable for both container–closure systems (Figures 5–7).
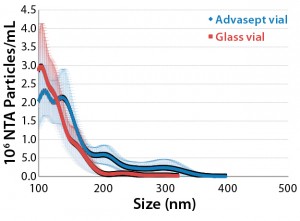
Figure 5: Mean submicron particle-count
data (nanoparticle tracking analysis) for MAb
formulations stored in glass and Advasept vials
for 12 months at 5 °C
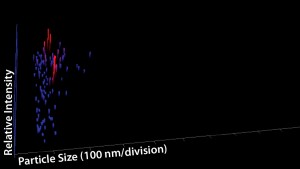
Figure 6: Particle size, relative intensity, and
concentration — three-dimensional (3-D)
graph for glass vial (12 months at 5 °C)
Â
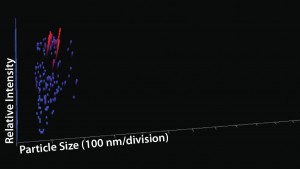
Figure 7: Particle size, relative intensity, and
concentration — 3-D graph for Advasept (12
months at 5 °C)
SDS-PAGE Analysis: In Figure 8, lane 5 is a nonreduced sample from a glass vial. Lane 6 is a nonreduced sample from an Advasept vial. In Figure 9, lane 5 is a reduced sample from a glass vial. Lane 6 is a reduced sample from an Advasept vial. Pre- and postfill and stability SDS- PAGE data show comparable heavy- and light-chain bands for both glass and Advasept vials.
cIEF Analysis: Capillary isoelectric focusing chromatograms at time-point 0 showed no comparable charge distribution between glass and Advasept vials (Figures 10 and 11).
Peptide Mapping: We sampled aged glass and Advasept vials at four months of 5 °C storage. For peptide mapping, we initially denatured and reduced the MAbs with dithiothreitol (DTT, also known as Cleland’s reagent), then alkylated them with iodoacetamide. After clean-up on a chromatography column, MAbs were digested with trypsin and AspN endoproteinase. We separated peptides with a UPLC column and used UV and MS detectors for analysis.
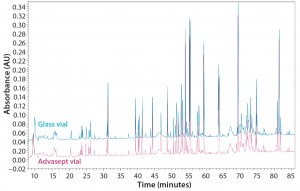
Figure 12: Magnified ultraperformance liquid chromatography with ultraviolet detection (UPLC-UV) chromatograms of trypsin-digested antibody rightway in a glass vial (bottom trace) and an Advasept vial (top trace)
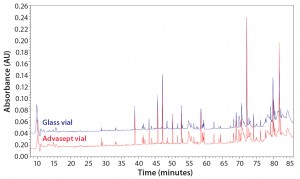
Figure 13: Magnified UPLC/UV chromatograms of ASP-N digested antibody rightway in a glass vial (bottom trace) and an Advasept vial (top trace)
As Figures 12 and 13 illustrate, both glass and Advasept vial samples showed no major difference in UPLC/UV chromatograms from both trypsin and AspN digestion, with 100% sequence coverage by MS detection. Deamidation levels observed with MS are comparable for both glass and Advasept vials (Table 4). We saw higher oxidation levels in glass vials than in Advasept vials, which could be attributed to surface interaction between MAbs and the glass surface causing more oxidation than in plastic Advasept vials.
Leachables: We stored our model MAb formulation samples for nine months at 5 °C before analyzing their contents for volatile, semivolatile, polar, and metal leachables using, respectively, gas chromatography with flame-ionization detection (GC-FID) and mass spectrometric detection (GC-MS), high- performance liquid chromatography with ultraviolet detection (HPLC-UV), and inductively coupled plasma mass spectrometry (ICP-MS) analytical techniques. The resulting leachables data indicate comparable profiles for MAb formulations stored in glass and Advasept vials — except for isopropyl alcohol. The isopropyl alcohol content was determined to be higher in glass vials (14 µg/mL) than in Advasept vials (1.2 µg/mL). We attribute that to cleaning procedures used for glass vials before filling of the model MAb formulation.
Equivalency Confirmed
Our results confirm compatibility of a model MAb formulation filled in both glass and Advasept container systems. This demonstrates that the Advasept BFS process and container system is suitable for protein therapeutics. Data from formulation samples before and after filling indicate that the slightly elevated temperature immediately at filling did not affect key characteristics of the model MAb.
Stability data related to aggregation, chemical degradation, affinity, and leachables indicate no significant differences between glass and Advasept container systems over the nine-month storage time we have analyzed to date. As demonstrated herein, the optimized BFS process had no impact on stability of a model MAb formulation. Thus, our results demonstrate that BFS is a viable and cost-effective method to produce aseptically filled biologic formulations.
Acknowledgments
We thank Kay Schmidt, Natasha Hults, Bill Hartzel, Gregory Bleck, Ian Collins, Keith Flood, and Thomas Luntz for their advice. This work was supported by Catalent Pharma Solutions.
References
1 CBER/CDER/ORA. Appendix 2 Blow-Fill- Seal Technology. Guidance for Industry: Sterile Drug Products Produced By Aseptic Processing — Current Good Manufacturing Practice. US Food and Drug Administration: Rockville, MD, September 2004; www.fda.gov/downloads/ Drugs/…/Guidances/ucm070342.pdf.
2 A Dedicated Environment. Pharmaceut. Manufact. Packing Sourcer May 2011; 96.
3 Goll AW. KB-0025-Jun12: Packaging Equipment — Blow/Fill/Seal (B/F/S) Technology. International Society of Pharmaceutical Engineering: Tampa, FL, June 2012; www. natcotechnologies.com/pdf/lot2/ISPE%20 packaging-equipment-blow-fill-seal.pdf.
4 Reynolds G, Paskiet D. Glass Delamination and Breakage: New Answers for a Growing Problem. BioProcess Int. 9(11) 2011: 52–57.
5 Sharma B, et al. Technical Investigations Into the Cause of Increased Incidence of Antibody-Mediated Pure Red Cell Aplasia Associated with Eprex. EJHP May 2004: 86–91.
6 Villabos AP, et al. Interaction of Polysorbate 80 with Erythropoietin: A Case Study in Protein–Surfactant Interactions. Pharm. Res. 22(7) 2005: 1186–1194.
7 Boven K, Bader F, van Regenmortel M. Immunogenicity of Biopharmaceuticals: An Example from Erythropoietin. BioPharm Int. August 2005: 36–52.
8 Sun L. Protein Denaturation Induced By Cyclic Silicone. Biomaterials 18(24) 1997: 1593–1597.
9 Jones L, Kauffman A, Middaugh C. Silicone Oil Induced Aggregation of Proteins. J. Pharmaceut. Sci. 94(4) 2005.
10 Bartzoka V, McDermott M, Brook M. Protein–Silicone Interactions. Adv. Material 11(3) 1999.
11 Markovic I. Challenges Associated with Extractables and/or Leachable Substances in Therapeutic Protein Products. Amer. Pharm. Rev. 9(6) 2006: 20–27.
Corresponding author Dipesh Shah, PhD, is a senior development engineer at Catalent Pharma Solutions, 2210 Lake Shore Drive, Woodstock, IL 60098; 1-815-206–1220. Gregory T. Bleck, PhD, is global head of R&D for Catalent Biologics (gregory.bleck@catalent.com), and Ian J. Collins is senior director of process development at Catalent Pharma Solutions (ian.j.collins@catalent.com).